For many, news of the first “breakthrough” COVID cases was alarming. But for scientists, it was expected—and presented an opportunity.
“We always knew there would be a certain number of people who develop infections even after being fully vaccinated,” says Robert B. Darnell. “What we didn’t know was what those cases would look like.” How severe would they be? Would some SARS-CoV-2 variants prove more adept at breaking through the vaccines’ protection than others? How would these cases impact the course of the pandemic?
As the global health crisis enters its second summer, the nexus of COVID research is shifting. We’ve come to understand the basics of how the virus infects host cells and replicates, and we’ve learned enough about the body’s immune response to create several good vaccines. But the world’s long-term relationship with this coronavirus, and other viruses like it, is still an open question.
One clue to how things will progress comes from surveillance within the Rockefeller community. Since January, mandatory weekly COVID testing of all on-site Rockefeller personnel has been conducted in-house by Darnell’s lab, using a saliva-based PCR test he and his colleagues developed (for more on the test, see Building a better COVID test). In addition to keeping the community safe, this program has produced a wealth of information, and it was among the first to document and explore what breakthrough cases look like at the clinical and genetic level.
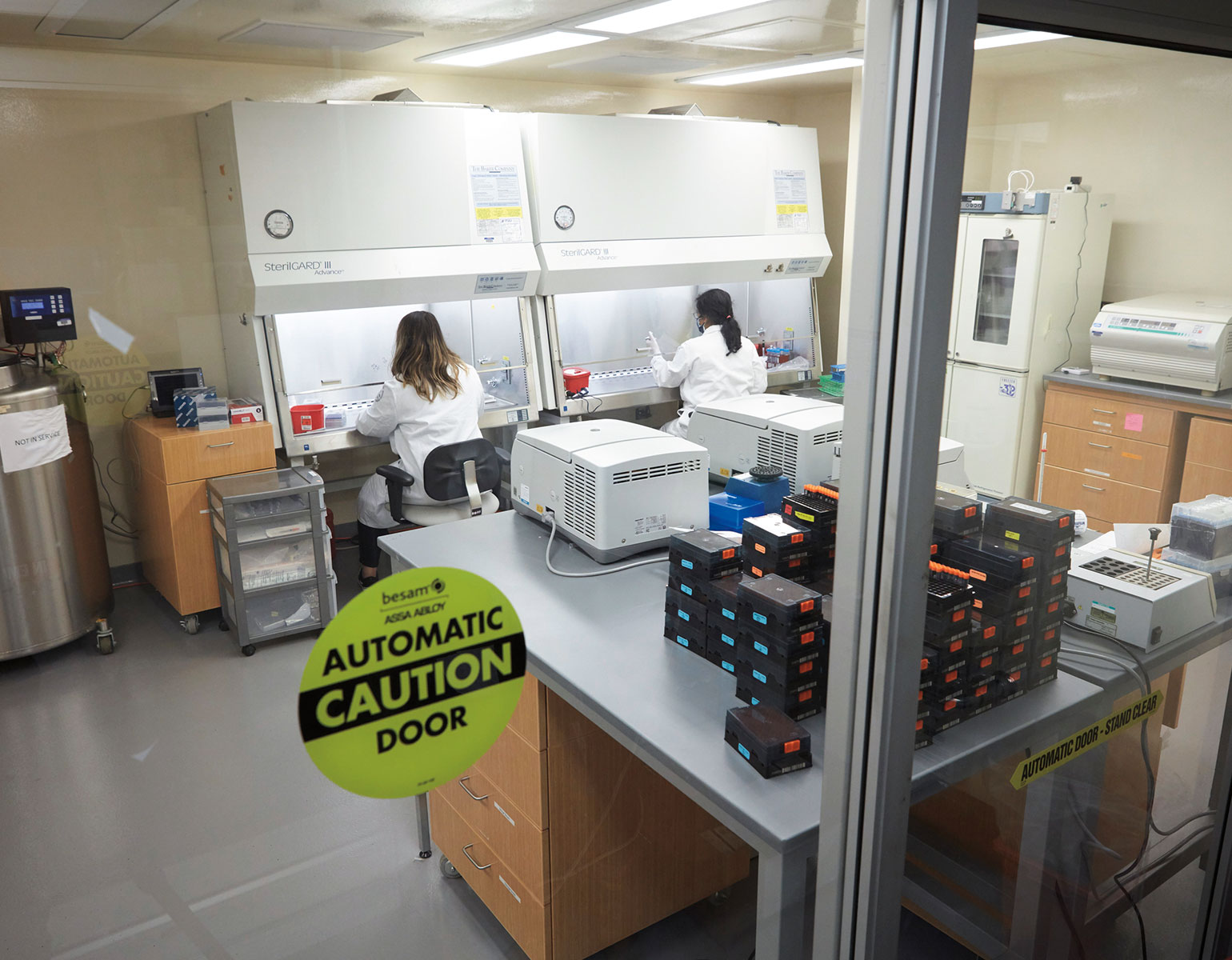
The results suggest reasons for both confidence and caution. The vaccines are holding up well to known variants, such as those originating in Brazil and the United Kingdom, and they prevent severe disease. But even a highly successful vaccination program doesn’t mean the end of COVID.
“Based on what we’ve seen, routine testing of any individual with flu-like symptoms, or those who have had contact with a positive case, will remain an important tool to prevent the spread of this disease for some time,” says Darnell, who is Robert and Harriet Heilbrunn Professor.
Meanwhile, pursuing treatments for COVID remains as important as ever. Monoclonal antibodies have shown exceptional promise over the past year, and one version developed at Rockefeller—a combination of two antibodies originally isolated from COVID patients who successfully fought off the infection early in the pandemic—entered clinical trials this January.
Similar antibody-based drugs have been used experimentally in thousands of COVID patients, and these drugs help stop the infection in its early stages before it progresses to severe disease. The cocktail developed by Michel C. Nussenzweig, the Zanvil A. Cohn and Ralph M. Steinman Professor, and his collaborators including virologists Paul Bieniasz and Theodora Hatziioannou, recently licensed to Bristol Myers Squibb, is designed to help minimize the risk of the virus mutating and developing resistance to the therapy.
Scientists are also pursuing new antiviral drugs that, similarly to broad-spectrum antibiotics, might be effective against multiple pathogens. A group led by Nobel Prize–winning virologist Charles M. Rice, the Maurice R. and Corinne P. Greenberg Professor in Virology, mapped a network of more than a hundred human proteins that SARS-CoV-2 hijacks as it takes over a cell’s replication machinery. One of them, a little-known protein called TMEM41B, stands out for its use by four different coronaviruses as well as by viruses that cause Zika, yellow fever, and other diseases. The team is investigating ways to disrupt TMEM41B’s ability to support an infection.
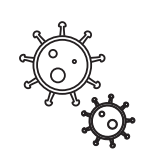
Data
There are many thousands of SARS-CoV-2 variants, and over 2,600 distinct lineages have been discovered so far. Four are considered “variants of concern” by the CDC.
Other researchers are studying how the virus impacts lung cells specifically. Because SARS-CoV-2 first enters the body via the lungs, its interaction with cells in the airways and alveoli is what allows it to establish a foothold in the body. A team led by Ali H. Brivanlou, Robert and Harriet Heilbrunn Professor, has used stem cell technology to produce tissue that mimics lung buds, the embryonic precursor to mature lungs (see Synthetic micro lungs). Beyond providing a realistic model to investigate the mechanisms of viral infection, the method can quickly produce vast amounts of lung tissue for drug-screening purposes.
As the pandemic evolves, so do our questions. What does the immune response to SARS-CoV-2 look like months or a year after infection? How does vaccination impact people who have already been infected? How well do our antibodies adapt to deal with the emerging variants of the virus? Bieniasz and Hatziioannou are studying the shifting relationship between our antibodies and the virus. Working with Nussenzweig, their team has found that in those who recover from COVID, the immune system retains a memory of the coronavirus, building a long-lasting defense in which antibodies are continually refined and improved.
What’s more, their work suggests that vaccination further boosts the neutralizing power of antibodies: Individuals who receive vaccines after having recovered from COVID should enjoy high levels of protection, even against emerging variants they haven’t yet encountered. Vaccinated individuals who haven’t been exposed to the virus, however, retain some vulnerability to the variants, their work shows.
“It’s a complex situation,” Bieniasz says. “And it suggests that vaccines may need occasional updates in the future to keep up with the mutating virus.”