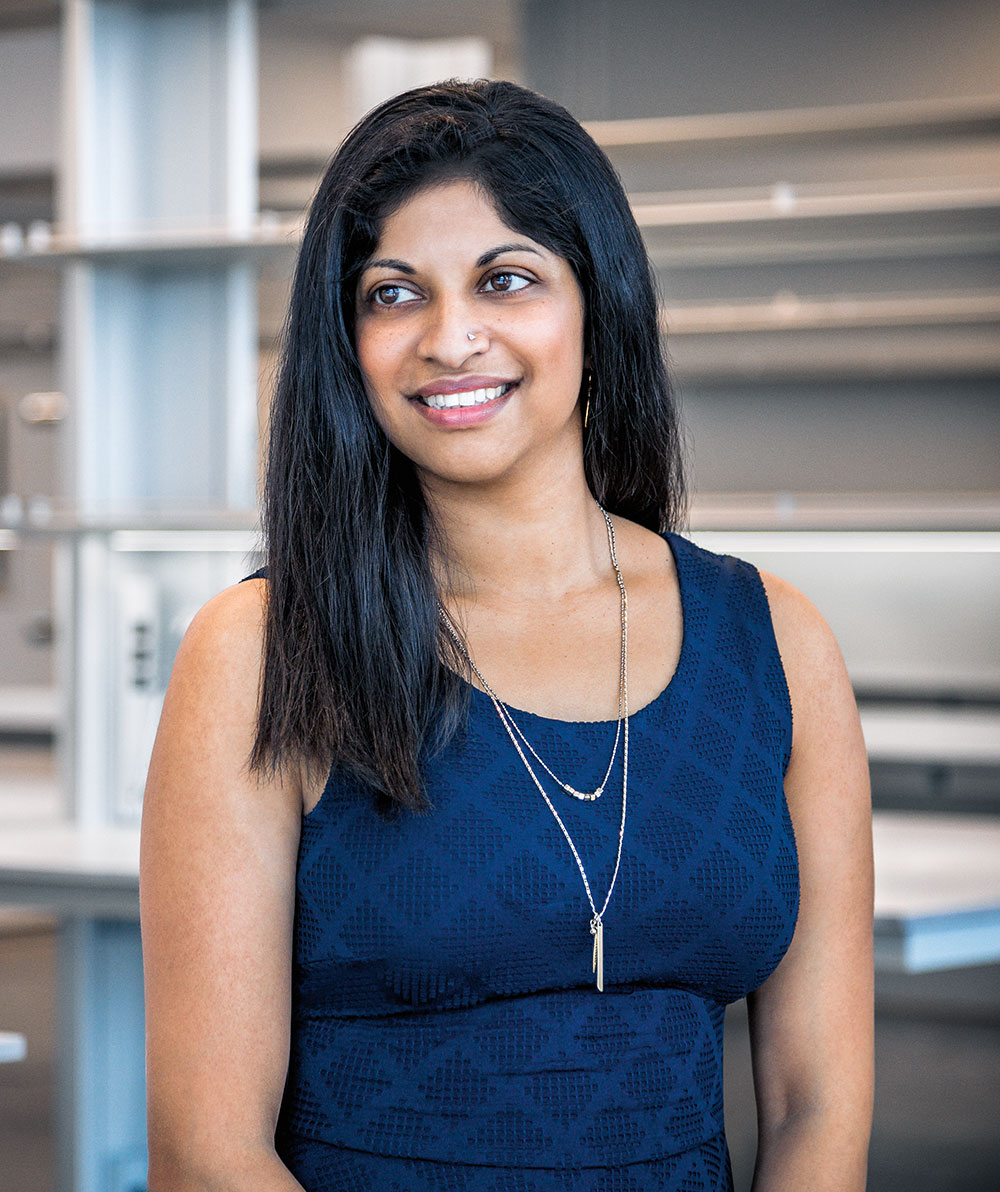
Becoming a scientist
Priya Rajasethupathy
Our understanding of memory is being overwritten. Meet the neuroscientist whose new lab is rethinking how our brains handle the past.
By Alexander GelfandAsk her how she came to science, and the memories come flooding back. Priya Rajasethupathy recalls a youthful fondness for chemistry and biology. She talks about community service, tutoring elementary school students. She describes how her father emphasized engineering—the best path toward developing quantitative thinking and job security, he believed—and how as a result she took a lot of math and computer science. She speaks of the intellectual thrill she had in her first college lab, and of the influence of her graduate and postdoc mentors.
And then she drops a bomb: you shouldn’t necessarily believe her. The next time you ask, her memories could be entirely different.
Rajasethupathy’s memories, like all memories, are malleable and subject to constant revision. As a neuroscientist who is dedicating her career to understanding how memories are recorded, stored, and retrieved in the brain, Rajasethupathy knows this better than most. And she knows why memories are so hard to pin down: To remember things, we rely on a series of biological processes that are constantly writing, retrieving, and rewriting our recollections, shifting them between neurons and passing them back and forth from one area of the brain to another.
“There’s an old model of memory in which a discrete experience is plucked from a fixed spot in the brain’s filing system when it’s needed,” Rajasethupathy says. “And there’s a newer one that suggests a far more dynamic decision-making process. We now see vast numbers of interacting neurons, spread across various brain regions, accumulating evidence and feeding each other bits of information before ultimately arriving at a remembrance—there’s a thought process you go through.”
Her research has shown that while a specific memory may seem stable, the assemblage of neurons and circuits that conjures it is in fact constantly changing and evolving. According to this model, every time you remember something, you are giving your brain the opportunity to make refinements. In other words, the memory process is less like the Encyclopedia Britannica and more like Wikipedia: constantly evolving and full of facts whose accuracy comes with no guarantees.
Rajasethupathy’s journey from aspiring engineer to physician to neuroscientist—as her brain presently portrays it—is in many ways a traditional one. She went to good schools, got good grades, made connections, followed her instincts, and had good luck along the way. From her days as a public school student in upstate New York to her studies at Cornell, Columbia, Stanford, and finally to her current job at Rockefeller, where she runs her own laboratory, Rajasethupathy has succeeded in a notoriously competitive field.
As a teenager, Rajasethupathy spent most of her free time volunteering: she tutored elementary school students in her hometown of Brockport, a village on the Erie Canal; worked at soup kitchens in inner-city Rochester, 20 miles away; and visited patients in hospitals.
“My whole life was really about working with people,” she says.
As an undergraduate, however, Rajasethupathy became drawn to biological entities of a much smaller variety. For her thesis, she set about trying to identify aptamers—short stretches of DNA or RNA—that may provide clues toward therapeutic compounds for epilepsy. She was soon consumed by the task, which combined the intellectual thrill of basic science with the prospect of improving the lives of actual patients.
Then, after leaving Cornell, and before pursuing her M.D., Rajasethupathy spent a year working on computational models of memory at the National Center for Biological Science in Bangalore, India, some five hours northeast of the village where her father had grown up. Here, she identified similarities between biochemical and transistor-based switches, suggesting how individual neurons and synapses store information.
The project allowed her to take full advantage of her early training in computer science, and it marked a turning point in her life: Though she still spent her free time doing community service and exploring the clinical side of neuropsychiatric disease, she found her attention turning ever more toward research.
Memory doesn’t operate in isolation; rather, it is closely tied to other aspects of cognition such as motivation and attention (try memorizing a phone number without focusing on it). As a result, you can’t really understand one without understanding the others. Exploring the basis of memory may also help elucidate the underlying causes of complex neurodegenerative and neuropsychiatric conditions, such as Alzheimer’s disease and attention deficit hyperactivity disorder, in which attention, emotion, working memory, and executive function are strongly intertwined.
And this understanding, in turn, could yield novel treatments for them.
Rajasethupathy’s work in Bangalore did not address any particular disease, or even answer any questions about how memory works. But it did raise some new ones.
Having just finished a highly theoretical computational project, for her Ph.D. work at Columbia, Rajasethupathy decided to dive into the nitty-gritty of how memory works at the molecular level. To do so, she needed sea slugs.
As it turns out, slugs from the genus Aplysia are the perfect creatures for this type of work. Each has just 20,000 neurons in its brain, visible to the naked eye and conveniently clustered in 10 well-defined regions. Rajasethupathy embarked on a project to find out how information is stored in individual synapses—asking how, in effect, the fleeting chemical signals that one neuron transmits to another can be inscribed in memory for a lifetime. Working with her Columbia mentor, Nobel laureate Eric Kandel, as well as with Rockefeller’s Thomas Tuschl, she discovered that a special kind of RNA molecule can migrate from a synapse to the nucleus of a neuron, permanently altering its DNA. The finding hinted at more permanent mechanisms for memory inscription.
The discovery that brain regions work together to store and retrieve memories would become the basis for her new lab.
At Stanford, Rajasethupathy traded slugs for mice in order to conduct behavioral experiments. How, she asked, does memory emerge from the interactions between different parts of a complex mammalian brain? By training mice to navigate virtual reality environments in which different rooms contained either rewards (a bit of sugar water) or threats (a sudden blast of air), and imaging their brains during their behavior, Rajasethupathy was able to trace the activity of clusters of cells in different brain regions as the animals created, stored, and retrieved memories.
In so doing, she discovered a previously unknown connection between the hippocampus and the prefrontal cortex, a part of the brain responsible for higher cognitive functions such as decision making and analytical thinking. She further demonstrated that this newfound neural circuit plays an important role in memory retrieval. This discovery—that brain regions work together to store and retrieve memories—would become the basis for her new Rockefeller lab.
In the fall of 2015, Rajasethupathy was ready to strike out on her own—to build her own lab after more than a decade of working in others’. It’s an enormously competitive undertaking: At Rockefeller she was one of 452 applicants.
Unlike many institutions, which conduct searches one at a time (a neuroscientist working on memory has just retired, so it’s time to find a new one), Rockefeller conducts an annual open search in which applicants from any bioscientific specialty are invited to apply. The university-wide search committee chooses the most promising scientists regardless of field. Some years, no one makes the cut, and in other years, there may be as many as four or five. Dozens of faculty members participate in the process, representing all major areas of study.
In Rajasethupathy’s case, there was little dissent.
“Her work was consistently innovative, even from the time she was a graduate student,” says Charles D. Gilbert, the Arthur and Janet Ross Professor. “Beginning in her postdoctoral work, to the present day, she has applied cutting-edge techniques to study important problems in neural systems research.”
Despite offers from other institutions, it was also an easy choice for Rajasethupathy, who was impressed by both the scientific excellence and the collegial and collaborative spirit on campus.
“You’re seen as a real person here,” she says. “At every level, you feel like people care about the decisions you make and what you’re trying to do.”
Letters were signed, boxes were packed. And on March 1, 2017, Rajasethupathy showed up in an empty Flexner Hall laboratory for her first day of work as an assistant professor.
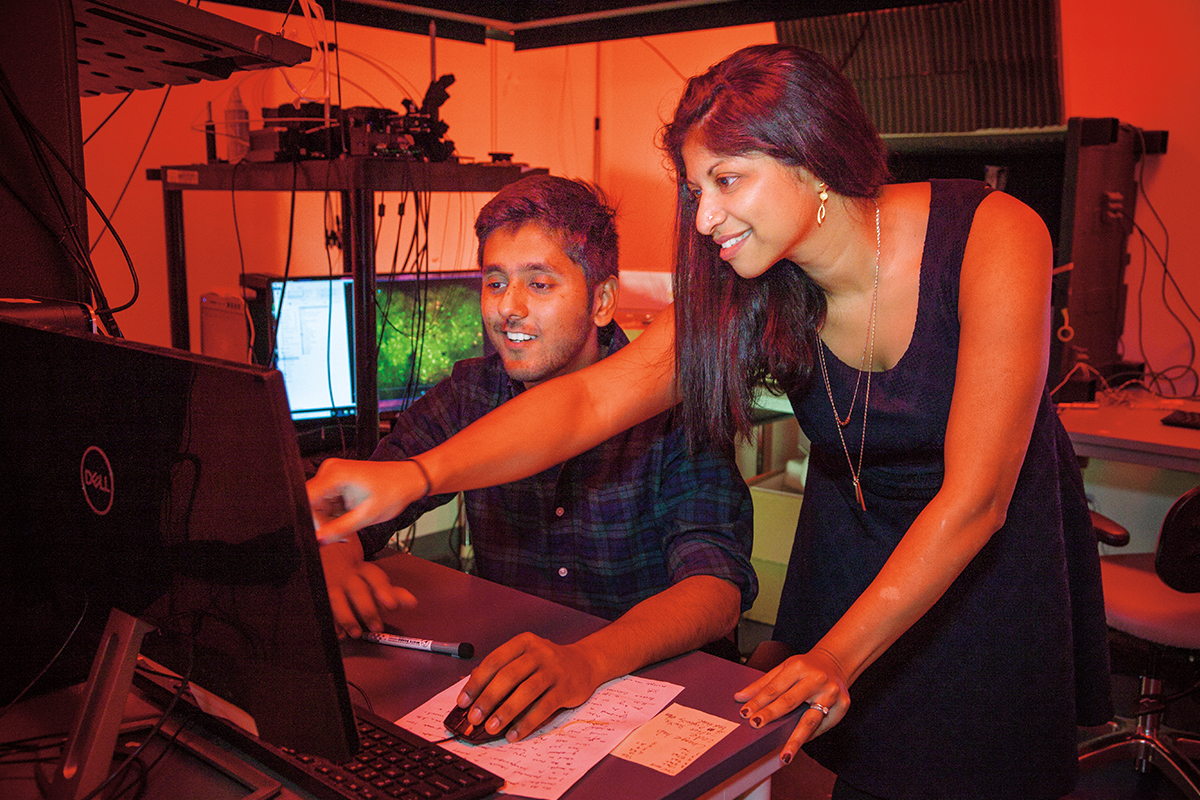
The thing that nobody tells you about starting a new laboratory is that it’s nothing like starting a new job. It’s more like building a new company. There are people to hire, students to recruit, budgets to manage, equipment to buy, supplies to order, and a million decisions to make. You’re expected to be a manager, mentor, editor, technician, engineer, accountant—and also a scientist.
“You’re completely ill-equipped to do this job when you start,” Rajasethupathy says with a laugh. “You come to really appreciate the luxury that students and postdocs have to focus on their own projects and devote all their time to science.” At Rockefeller, administrators offer much support, and there are helpful colleagues to provide advice, but the responsibility to convert a winning research proposal into a functioning laboratory still rests on the new recruit.
It didn’t take long for Rajasethupathy to find her groove. Within a year, she had realized that the best part of the job is the very thing that got her started down this road in the first place: service to others.
“Number one on my list now is to mentor my students and get them to love and appreciate science,” she says.
She has also recommitted herself to community service through the university’s outreach programs: engaging with undergraduates, high school students, and even the kids in the university’s Child and Family Center, where her children attend day care.
Her scientific priorities have evolved as well. In the past, Rajasethupathy focused largely on fundamental research. But increasing encounters with patients, donors, and those who have witnessed the impact of mental illness and brain disease—combined with the acknowledgment that taxpayers fund much of her research—have prompted her to think more about projects with significant medical applications.
Traditionally, neuroscience has relied on inbred or genetically engineered animals that lack the genetic variability of human populations. Rajasethupathy and her team are therefore using wild-derived strains of outbred mice to capture that variability. This allows them to observe the natural development of cognitive deficits associated with specific human disorders, including Alzheimer’s and ADHD.
By analyzing the animals’ genomes, Rajasethupathy and her collaborators at Cornell hope to identify novel genes and gene pathways, as well as the resulting neural-circuit features, that drive these cognitive traits. That, in turn, will lead to a better understanding of the molecular pathways and neural circuits involved in complex human diseases: pathways and circuits that could provide new avenues for treatment, whether through novel drugs that latch on to particular molecular targets or nonpharmacological therapies such as deep-brain stimulation that directly target the neural circuits.
Describing her work to a standing-room-only crowd of Rockefeller scientists last December, Rajasethupathy discussed her finding that brain cells known as hub neurons recruit other neurons from different areas of the brain to help form and retrieve memories. This discovery hints at why memories are so stable and can be recalled so rapidly; but it also suggests that the high metabolic activity of these hub neurons, which serve adaptive and integrative functions for memory, may make them particularly vulnerable to destruction during neurodegenerative disease.
She also outlined her ongoing efforts to understand the give-and-take that occurs between the hippocampus and other brain regions, work that could in time provide insights into the physiological processes of forming and retaining long-term memories, and help explain how these processes sometimes go awry.
If she was nervous delivering her first, formal research update, it didn’t show. On vivid display was not only her lab’s recent data, laid out in striking multicolored slides, but also Rajasethupathy’s unmistakable love of her work and infectious sense of humor. Her audience reciprocated, asking probing questions and volunteering helpful advice.
“Their support reminded me of how thankful and fortunate I am to be here,” she says. “Joining Rockefeller is the best decision I ever made.”
Or at least, that’s how she’ll remember it.