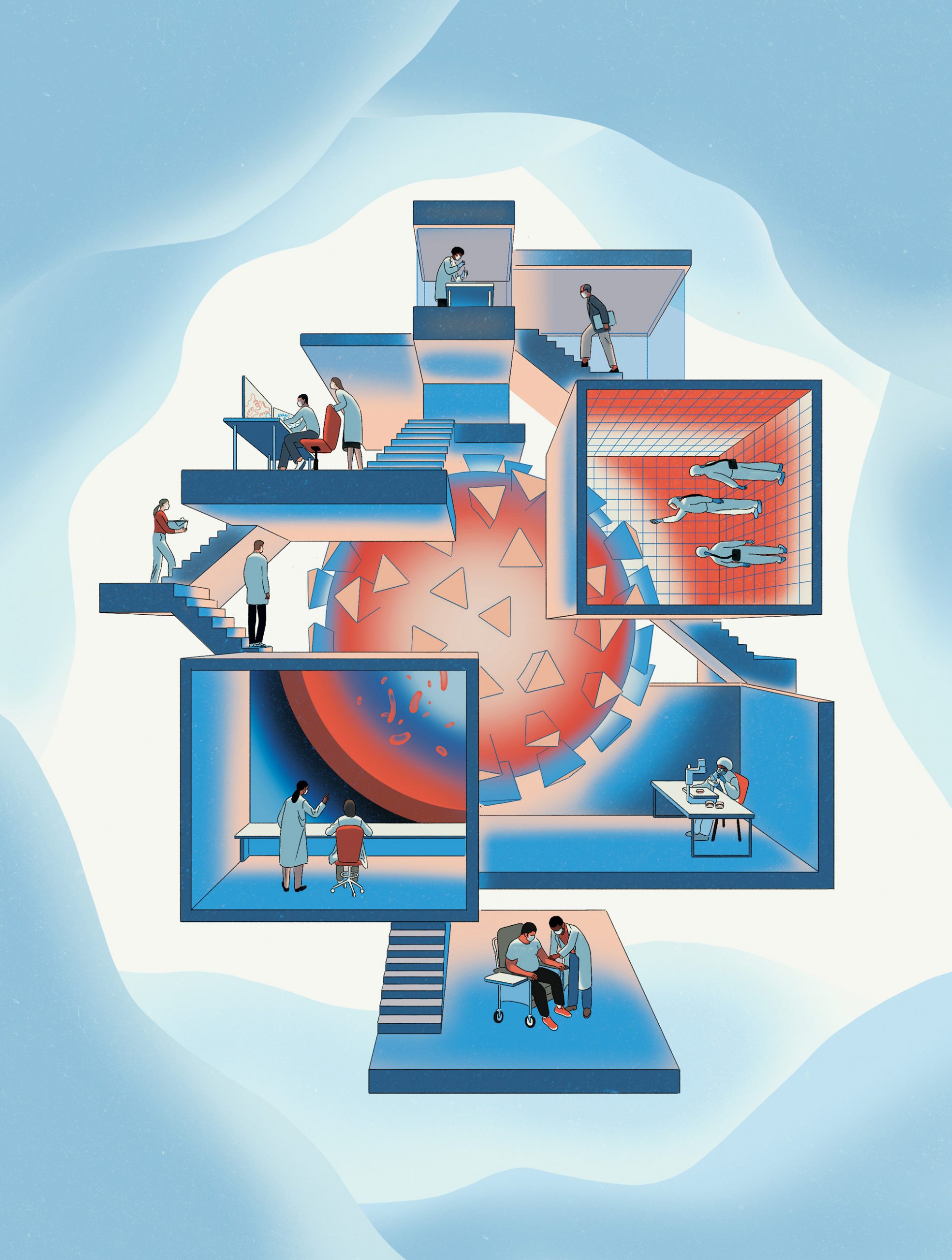
Feature
Inside the response
Researchers everywhere have united to address a singular, urgent problem—a virus that is devastating the world. Their approach is to leave no stone unturned and employ every technique in the 21st-century science playbook.
By Bahar GholipourIt was the end of March. The Rockefeller campus was quiet but for the sound of birds and the lone crackle of a lab-waste cart, dragged along an empty hallway. In the gardens, blossoms were beginning to emerge but humans were a rare sight. The scene mirrored the larger surroundings, a city of eight million that seemed to have suddenly frozen in time, save for ambulances zipping by.
Yet despite this seeming tranquility, some labs were bustling. Virtually overnight, teams were putting together entirely new projects and beginning to conduct experiments under the added complication of social-distancing guidelines, with one crucial goal: to help alter the course of a pandemic that had brought the world to a standstill.
98,941Number of scientific papers on COVID-19 published in the first nine months of 2020.
It usually takes years or even decades for basic-science insights to ripen into medical innovations, but in this age of the COVID-19 pandemic, a new mode of discovery is emerging. Unified in their purpose, scientists are collaborating across disciplinary and national boundaries in a tremendous race to understand a new virus. Their joint scope is vast: Some lines of investigation could help COVID-19 sufferers in the near future; others take a longer view, seeking to understand lasting medical effects of the disease and preparing for new viral outbreaks yet to come. The projects aim to attack the virus from every angle, understand how it behaves and thrives, figure out why some people get more severely sick from it than others, and devise new methods and tools to assess potential treatments.
Considering how much death and destruction SARS-CoV-2 has already caused, the challenges ahead are daunting. Yet there are good reasons for optimism given how far bioscience has come since the last time humanity was hit by a pandemic of this intensity. With the 1918 flu, it took 15 years just to identify the culprit pathogen. The disease was widely believed to be bacterial until Richard Shope, a Rockefeller virologist, isolated the H1N1 influenza virus in 1933.
Scientists are once again faced with a mysterious pathogen that has so far defied treatment. This time, however, they are equipped with tools of extraordinary power and precision, and knowledge garnered over decades of intensive study. Their challenge is to redirect their expertise—whether in immunology, genetics, biochemistry, or other fields—to a single problem, a diabolical virus that has killed over a million people and devastated societies worldwide.
Here we’ll take a look at three rapidly unfolding COVID-19 research projects born in the midst of a state-wide lockdown.
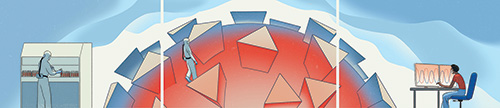
From one pandemic to another
If Michel Nussenzweig expected the new year to be busy, it was mainly because of a virus that’s been around for nearly 40 years. In January, the university had handed off the yields of a decade-long HIV research project to a pharmaceutical company to collaborate on developing a new, landmark drug based on HIV antibodies. In no time, however, a new challenge was on Nussenzweig’s desk: The lab needed to clone more antibodies, this time for an emerging coronavirus that day by day was looking more like a severe threat with pandemic potential. The idea was to quickly develop treatments that, like the new HIV drugs, would harness the immune system’s natural virus-fighting powers—specifically by identifying and copying exceptionally potent antibodies found in patients who had successfully recovered from the infection.
“This will happen again, for sure. We need more emphasis on infectious disease research so that next time the scientific community can respond even faster.”
Nussenzweig, the Zanvil A. Cohn and Ralph M. Steinman Professor, pushed off at once, working with immunologists, virologists, and medical scientists spanning several labs within and beyond Rockefeller. Step one was to get their hands on hundreds of SARS-CoV-2-targeting antibodies and to sift through that stockpile to identify those variants that neutralize the virus most effectively by blocking its entry into cells.
It was a needle-in-a-haystack proposition since most antibodies are, at best, capable of only a blunt, imprecise attack on the virus, like hitting it with a baseball bat. Potent neutralizing antibodies, on the other hand, are what Nussenzweig describes as “precision guided missiles with nuclear warheads”—capable of delivering a decisive and fatal blow to the virus. Their potency means that, instead of having to mimic the whole spectrum of antibody responses, researchers can manufacture just one or two antibodies in large quantities, to be used as a drug that people can take during the early stages of infection. This antibody injection would, hopefully, give the immune system a head start in fighting the virus before it can gain a foothold.
To find those prized antibodies, the scientists needed blood from people who had successfully fought off the infection—and they needed it fast.
Fortuitously, Jill Horowitz, a member of Nussenzweig’s team, lives in New Rochelle, a New York City suburb where the country’s first sizable outbreaks emerged. Horowitz immediately got to work recruiting recovered patients willing to help: She arranged a town hall in the synagogue where the virus had struck, she went on the local radio, she handed out flyers in the shopping mall. “In that early stage, it was crucial to recruit as many people as possible as quickly as possible,” Horowitz says. After that kick-start, the recruitment team led by Rhonda Kost, in The Rockefeller University Hospital, reached recovered individuals from across the greater New York City area.
Their efforts paid off: In a few short weeks, flocks of COVID-19 survivors visited campus to donate blood plasma, from which Nussenzweig’s team extracted antibody-producing immune cells using a tried-and-true technique they had developed for their HIV research. (Read more about the lab’s work on HIV in “An end in sight.”)
Next, the researchers needed to find which of these individuals produce the exceptional antibodies—and here they came up against another roadblock. The procedure normally entails growing the virus in the lab, bathing it with each antibody, and assessing which antibody has the best neutralizing capacity. It’s a labor-intensive and time-consuming procedure, especially when you’re dealing with a virus as contagious as SARS-CoV-2, which must be handled in a biosafety level 3 laboratory (see “Top-tier biosafety”). To circumvent this step, Nussenzweig turned to his colleagues Paul Bieniasz and Theodora Hatziioannou, who swiftly came up with a work-around: a faux coronavirus that serves as a stand-in for the real thing in lab experiments but is much more convenient to work with.
This pseudo-coronavirus is made up of a different virus, typically vesicular stomatitis virus, that has been rendered unable to replicate. It is then tweaked to express the SARS-CoV-2 spike protein, which is what the coronavirus uses to gain entry to cells. “We basically decorate another virus with the spike protein and use it as a proxy for SARS-CoV-2 infection,” Hatziioannou explains. Working with virologist Charles M. Rice, the Maurice R. and Corinne P. Greenberg Professor, she and Bieniasz found that, for their purposes, the pseudo-virus worked just as well as the real one (see “The faux coronavirus,” above).
A picture of the immune system’s response to SARS-CoV-2 soon started to emerge. The scientists found that the spectrum of plasma antibodies varied widely among infected people, but about one percent of donors had sky-high levels of antibodies with neutralizing superpowers.
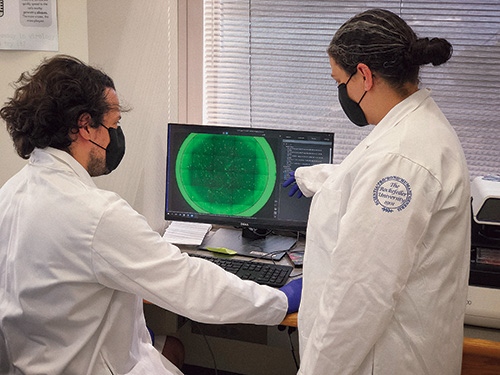
“To find individuals with such potent antibodies was a great relief,” says Davide Robbiani, a research associate professor in Nussenzweig’s lab. “We expected to see everyone respond differently, but there was no guarantee that we would find exceptional responders like we had in the past with HIV-1. And the fact that they do exist among people who recovered from COVID-19 was positive news for the development of antibody-based drugs.”
By early May, merely a month after the city went into lockdown, the team had zeroed in on three unique antibodies that had all the features of a good drug candidate. Although they came from different patients, these antibodies share a key feature: They bind to a critical region of the spike protein, the so-called receptor-binding domain, presumably blocking it from docking onto the host-cell receptor to gain entry. And they could block the virus even at extremely low concentrations.
“Never before for any infectious disease have we been able to decipher the root causes for such a large proportion of severely affected patients.”
What’s more, these antibodies bind to the receptor from different angles, suggesting that a combination of them could work together to put an airtight seal on the spike protein and make it harder for the virus to escape the therapy by mutating.
The summer months were a whirlwind of advancing the three antibodies down the development path, where steps normally done in sequence were instead happening in parallel. As animal studies were conducted, for example, the antibodies were being prepared for the human trials, and plans and infrastructure were put in place for producing huge quantities of the antibodies, standing by to be deployed if the clinical trials succeed.
“It is an extraordinary effort—and it’s gratifying to be able to condense all the expertise we gained over a decade of HIV-1 work and pour it into a much larger project, much faster,” says Marina Caskey, a clinical investigator who has been designing the human trials, both small ones conducted at the university and larger multi-institutional trials scheduled to begin in December in some of the country’s hot-zone areas.
This fast-tracked experience in antibody research and development might also set the stage for future responses to emerging pathogens. “This will happen again, for sure,” Nussenzweig says. “It’s now clear that we need to put a lot more emphasis on dedicated infectious disease research, so that next time, the scientific community can respond even faster.”
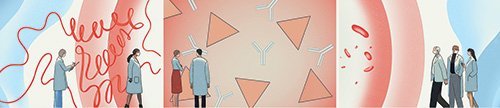
It’s not just the virus.
It’s also us.
While airports were deserted of human passengers, plastic vials containing human blood and DNA samples were being ferried around the world in record numbers. Many were collected from COVID-19 survivors who were relatively young and had no underlying conditions. When these patients ended up in hospital ICUs, they defied a common narrative about the disease: that symptoms would be mild in those who weren’t elderly or immune suppressed.
But on the seventh floor of The Rockefeller University Hospital, where hundreds of these blood samples would eventually arrive, researchers were not at all surprised. “We see this pattern in every single infectious disease we have studied,” says Qian Zhang, a research associate in Jean-Laurent Casanova’s lab. “There is always a subgroup of people who get severely sick.”
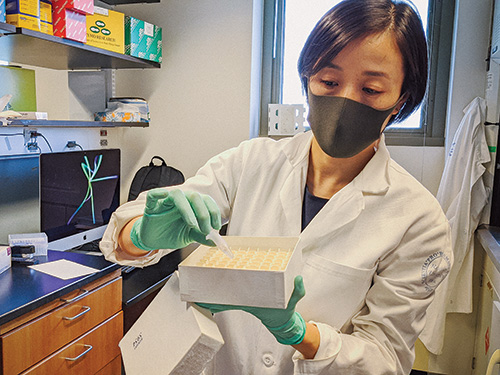
Zhang and her colleagues believe that the samples they’ve collected from COVID-19 outliers might hold important insights—clues to understanding the disease that scientists won’t necessarily find by examining the average patient population. In recent decades, knowledge gained from the lab’s study of similar outlier cases has led to new treatments for mycobacterial disease, herpes simplex virus infections, and other infectious diseases. And in all these cases, the reason the disease became unusually severe could be traced to a traditionally overlooked place: the patients themselves.
“Infectious diseases are not just about the pathogen. There is always an interplay between the pathogen and the host’s immunity,” says Casanova, head of the St. Giles Laboratory of Human Genetics of Infectious Diseases.
His lab’s approach involves searching people’s DNA for alterations that cause subtle defects in the immune system—cracks that pathogens might exploit. In many cases, those who carry this kind of alteration won’t know it. They might be susceptible only to a specific pathogen—and as long as they don’t encounter that pathogen, the mutation will go unnoticed.
In early January, when Casanova first heard about an emerging infectious disease in China, he wasted no time looking for such ticking-bomb mutations. As many other scientists scrambled to shift their focus to the new and mysterious disease, he already had a plan—the same plan he has successfully applied to many other illnesses. “I immediately got in touch with colleagues in Asia and asked for samples from their most severe patients,” he says.
$2.2BNIH grant money awarded for COVID-19 research in the first nine months of 2020.
And just as quickly as the virus swept the world, Casanova’s project ballooned into an international collaboration, called the COVID Human Genetic Effort, spanning more than 30 labs and numerous collaborating hospitals around the world. Co-leading the group with Helen Su of the National Institute of Allergy and Infectious Diseases, Casanova has since enrolled thousands of patients who were hospitalized for COVID-19. “The approach is to try to enroll as many patients as possible, in as many hospitals as possible,” Casanova says. “That’s the only way to detect a signal when you are searching for a rare genetic variation.”
Recently, the team published two major discoveries pinpointing causes of severe COVID-19 in subsets of cases. Among the several hundred patients studied so far, the researchers have found that about four percent carry genetic mutations affecting type 1 interferons, proteins crucial for the immune system’s response to the virus. Normally, cells secrete these proteins at the start of an infection, but the researchers detected little or no interferons in the blood of patients with these mutations. Another 10 percent of patients were found to have misguided antibodies that were attacking not the virus but the immune system itself—specifically type 1 interferons.
Taken together, the results suggest that deficiencies in a specific immune mechanism are behind a significant number of COVID cases that become severe. “Never before for any infectious disease have we been able to decipher the root of the problem for such a large proportion of the severely affected patients,” Casanova says.
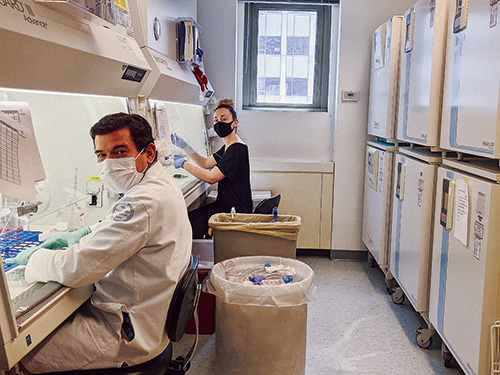
There is still more to learn. Because mutations that make people uniquely vulnerable to infectious diseases are rare, it will take time to gather conclusive data about the many causes of such vulnerabilities, Casanova says. Still, he is hopeful that even the early findings will have practical implications. For example, type 1 interferon already exists as a drug and could potentially, he says, benefit people who develop life-threatening COVID-19 but can’t produce it on their own.
Casanova’s team is also looking for mutations in a second group of people who seem to be resistant to SARS-CoV-2 infection, including spouses of infected individuals who test negative and remain symptom-free. “Whether a genetic alteration makes a person more vulnerable or more resistant to infection, it has something important to teach us about how the virus interacts with the immune system,” Casanova says. “And ultimately, such knowledge could lead to treatments for anyone who’s been infected.”
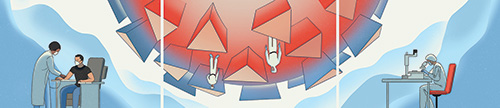
The chemistry conundrum
From a scientific point of view, SARS-CoV-2 represents more than a threat to humanity. It’s also a marvel of biochemistry: a mere strand of RNA wrapped in a protein shell that, despite its simplicity, is capable of preying on organisms as complex as ourselves. Its biological machinery may be slim, but it’s incredibly feisty.
“Coronaviruses are likely to show up more and more in the human population.”
Once the virus finds its way into human cells, it immediately gets to work replicating itself. A suite of enzymes and proteins works to read and write the SARS-CoV-2 RNA into thousands more copies; pack each copy into a new shell; and send the viral particles bursting out of the cell like confetti out of a popped balloon.
Disrupting any step in this process could potentially sabotage the virus’s replication scheme, and researchers around the world are now hunting for compounds that will glom on to critical viral enzymes and thwart their activity. Among them is Sean F. Brady, the Evnin Professor, who’s looking for such would-be drugs by surveying DNA from soil samples.
Dirt may seem like an odd place to look for solutions to the current catastrophe, but for Brady there is no better. For years, he has successfully exploited soil in preparation for a different crisis that, despite receiving far less publicity than COVID-19, poses an equally serious threat: the rise of antibiotic-resistant bacteria such as M. tuberculosis and C. difficile.
A spoonful of soil contains thousands of species of bacteria, which together produce a plethora of replication-slowing compounds—natural antimicrobials that the bacteria release as they compete with other microbes for nutrients and space. Brady has pioneered a unique method to find these chemical weapons by extracting bacterial DNA from soil, searching it for antimicrobial-producing genes, and reverse-engineering those compounds in the lab (read more about Brady’s approach in “Drugs from dirt.”)
At the outset of the COVID-19 crisis, Brady redeployed the same technique to search for bacterial compounds with antiviral properties. “The whole premise is that we go out to find nature’s solutions to microbial problems, whether it’s a bacterial infection or a viral one,” he says.
3,532Number of COVID-19 clinical trials launched in the first nine months of 2020.
To find potential antivirals, the team went back to their vast libraries of bacterial DNA extracted from soil, searching for signatures in the sequenced DNA that suggest a group of genes might make a certain type of molecule. The team is particularly interested in finding genes producing inhibitors of the RNA-dependent RNA polymerase, the key enzyme in SARS-CoV-2’s replication apparatus.
In the short few months since taking this new direction, the scientists have identified several compounds showing antiviral activity in lab experiments. They are also pursuing candidate drugs isolated not from soil bacteria, but from our own microbiome. “Just like bugs growing in the environment, the bugs that grow inside us make molecules that have structures similar to antivirals,” Brady says. “But are they truly antivirals? We don’t know yet.”
Elsewhere on campus, Tarun Kapoor, the Pels Family Professor is scheming to target another essential item in SARS-CoV-2’s replication toolbox: the helicase. Vital to all organisms, helicases are like molecular motors that move along strands of DNA—or in the case of coronavirus, RNA—unzipping them in preparation for the genetic information to be copied.
Like Brady, Kapoor hopes to find drugs for COVID-19 by leveraging decades of research in other fields. He worked on developing new drugs to stop cancer cells from replicating—a strategy he’s now adapting for SARS-CoV-2. His team has already identified the virus’s helicase and a suite of compounds that may be able to inhibit it. Both Kapoor and Brady are now working with Rice to test their compounds and identify those poised to stop viral replication.
SARS-CoV-2 is both a threat to humanity and a marvel of biochemistry, slim but feisty.
Success in any of these avenues could go beyond curbing the current crisis. In only the past 20 years, no less than three coronaviruses—SARS, MERS, and now SARS-CoV-2—have jumped from animals to humans, causing considerable suffering and economic damage, and there is no shortage of coronaviruses lurking in nature.
“What’s remarkable is that the helicase in SARS-CoV-2 is 99 percent identical to the helicase in the virus that caused the SARS epidemic in 2002,” Kapoor says. “Coronaviruses are likely to show up more and more in the human population, so learning how to target the replication machinery of this one could potentially prepare us for future versions.”