The humble wild cabbage has had one since 2016. A lowly gut bacterium has had one even longer. Now, courtesy of the Human Pangenome Reference Consortium—an international alliance whose leaders include Rockefeller’s Erich D. Jarvis—we Homo sapiens finally have one too: a genetically diverse pangenome that promises to dramatically increase our understanding of human disease and expand access to personalized medicine.
When the human genome was first released in 2003, scientists were already working to improve it. Over the next two decades, technology advances made it possible to fill in gaps and correct errors, but a substantial problem remained: Two-thirds of the DNA in the original reference genome came from a single person. As a result, many genetic variants found in non-European populations, such as people of African or Asian descent, weren’t included.
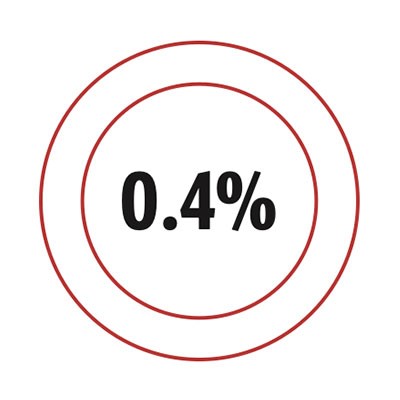
Data
The fraction of human DNA that varies from person to person.
This lack of representation can lead to biases in biomedical data that may in turn contribute to inequities and health disparities between different groups, Jarvis says. Among people with European ancestry, researchers have discovered countless genetic variants that predispose to specific illnesses, influence the severity of disease, or affect responses to particular drugs—knowledge that can provide powerful tools for physicians to diagnose diseases, predict health outcomes, and tailor treatments for individual patients. Such discoveries have yet to be made for populations whose variants were excluded from the reference genome in the first place.
The human pangenome that Jarvis and his colleagues have unveiled unveiled is a first step toward tackling these problems head-on. Obtained with DNA from 47 people from around the world, it reveals nearly 120 million new DNA data points. Most are related to so-called structural variations, genetic differences that arise when long stretches of the double helix are duplicated, deleted, or rearranged.
“Structural variations can have dramatic effects on trait differences, disease, and gene function,” Jarvis says. “There will be a lot of new discoveries to come that weren’t possible in the last 20 years.”