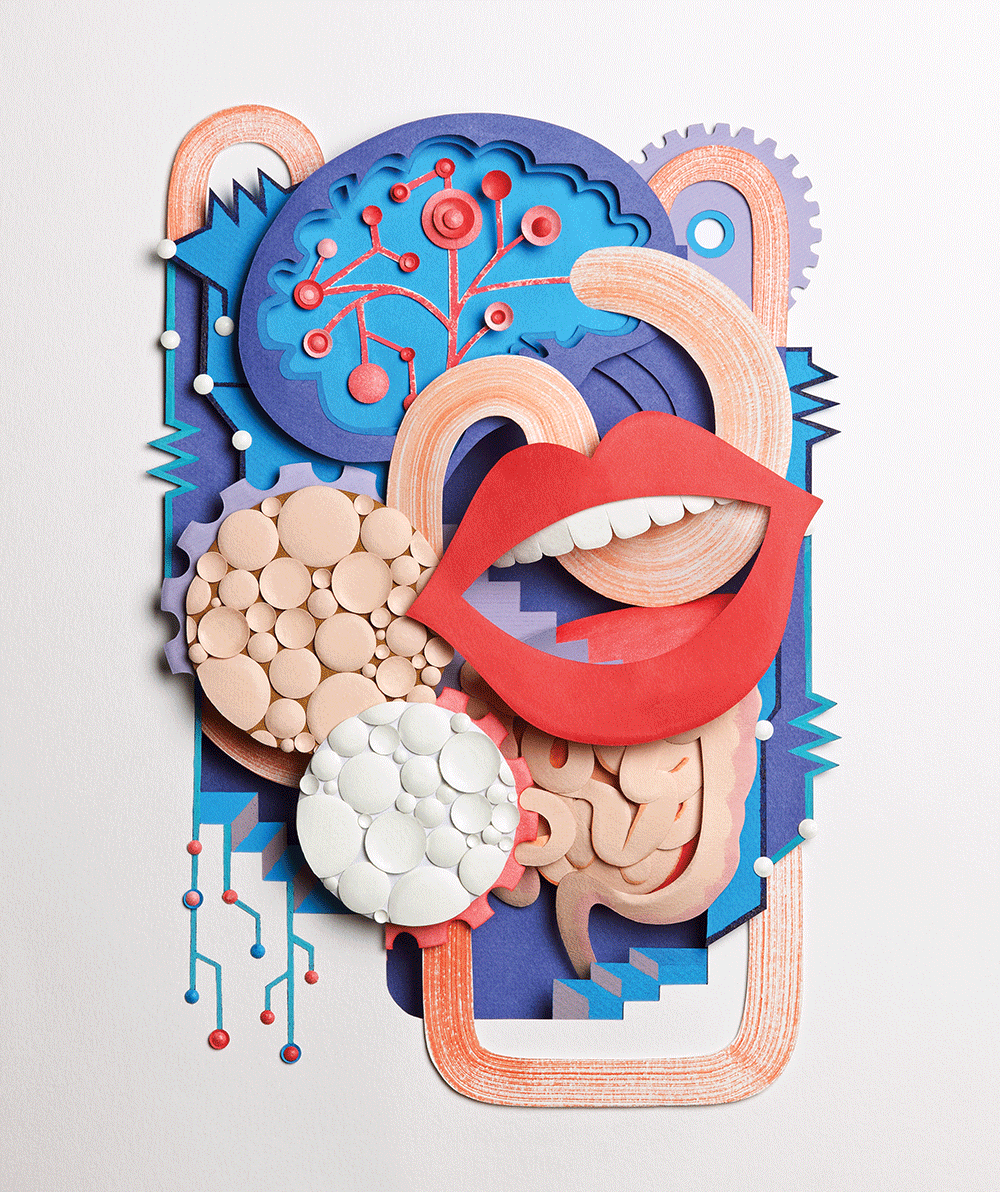
Feature
Will science end obesity?
First, it was a status symbol. Then, a flaw of character. Now we know it’s a biologically complex disorder whose mechanisms are just beginning to emerge.
By Alexander GelfandThe first pharmacological approach to weight loss was a total failure. Introduced in 1933, the metabolic stimulant DNP was pulled from the market in 1938 after it was shown to cause sudden death. Perhaps its toxicity shouldn’t have been too surprising: DNP had previously been mass-produced by the French as an ingredient for WWI munitions.
DNP wasn’t the last questionable weight-loss treatment, nor the first in a general sense. As ancient artworks attest, obesity has likely been around for as long as human culture itself. The word, which derives from the Latin obesus, meaning “having eaten until fat,” was coined in the early 1600s by a British physician, who recommended bathing in warm natural springs. If not terribly effective, at least his prescription sounded pleasant. In ancient Greece, Hippocrates advised patients to ingest herbal emetics and cathartics, “eat only once a day, … and walk naked as long as possible.”
Like so many who followed, the Greek physician was trying to help. It has long been known that, beyond a certain point, carrying excess weight can become a serious health concern. And for just as long, the history of obesity treatment had been a litany of failures. Curiously, even once the era of modern science arrived and physicians began to understand the outsize role that genes and other biological factors play in human health, the overweight were still stubbornly admonished to just eat less or burn more calories.
“Unfortunately, that remedy is more a symptom of a common misconception and no more effective today than when Hippocrates proposed it more than 2,000 years ago,” says Rockefeller neuroscientist Jeffrey M. Friedman, who in the 1990s essentially launched the field of molecular obesity research.
The simple act of eating is an extremely complex behavior: the execution of a fundamental biological need that’s influenced by culture, demographics, and economics. Having once been so rare it served as a sign of wealth and status, obesity has become significantly more prevalent since the 1980s, especially in developed countries: Here in the United States, some 100 million people are now considered to be clinically obese. At the same time, however, an equivalent number of Americans remain lean. How could that be? Why—even in the presence of a similar environment—do some people pack on the pounds while others don’t? And more importantly, why does excess weight lead to serious health complications in some people and not in others?
Science is beginning to find answers. Over the last four decades, Friedman and a network of researchers—some working together, others in parallel—have begun to unearth the genetic and molecular roots of obesity and eating-related behaviors, as well as the nature and function of fat and the crucial role played by various hormones in regulating appetite. Besides overturning conventional wisdom about the disorder, their work has led to the creation of a new class of blockbuster weight-loss drugs and the promise of an even wider array of safe, effective treatments to come.
Leptin, a key hormone
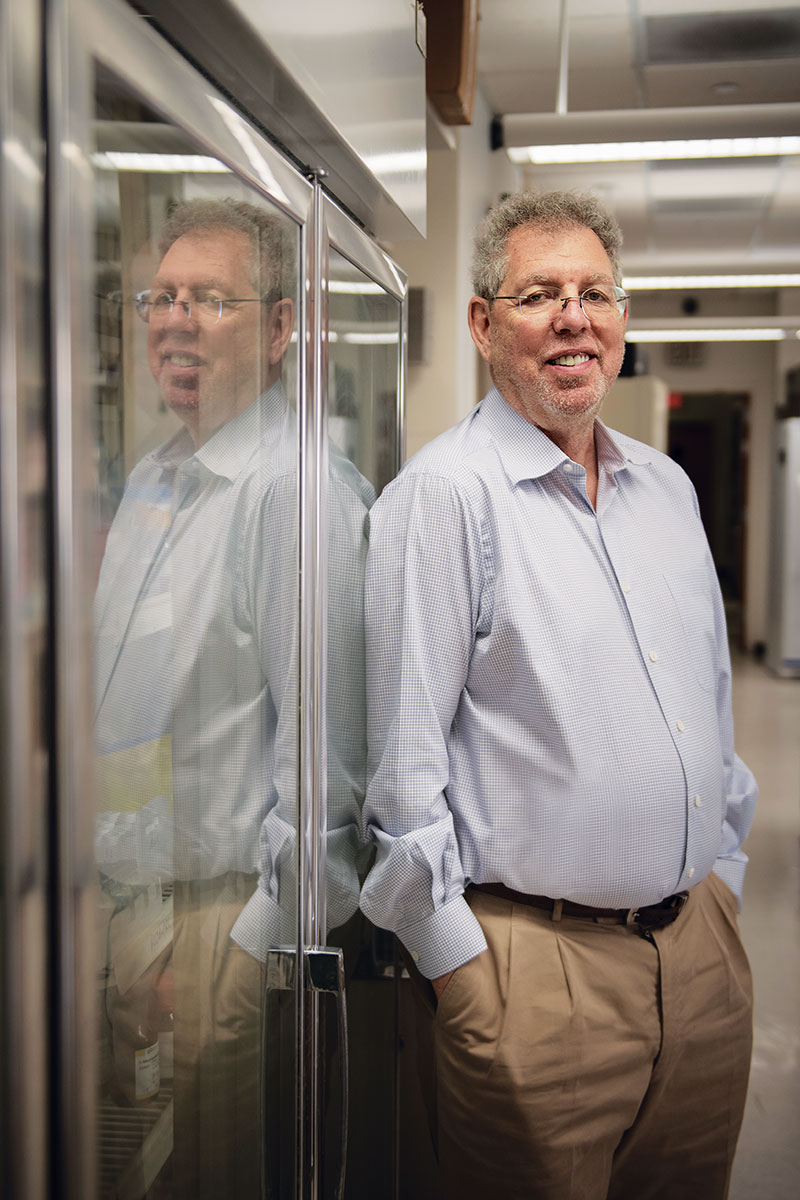
In 1994, Friedman discovered leptin, a hormone secreted by fat cells that plays a crucial role in regulating appetite and body weight. Because animals need leptin to stay lean, he named the hormone after the Greek word for thin, leptos. As head of the Laboratory of Molecular Genetics and Rockefeller’s Marilyn M. Simpson Professor, Friedman has since been tracing the neural circuits that govern food intake and leptin’s role in that process, work that has ultimately shed light on the mechanisms driving overconsumption and obesity. “I got into this,” Friedman says, “because it’s not understood how behavioral decisions are made in the brain, or even where they are made.”
To investigate such decisions, Christin Kosse, a postdoctoral fellow working in Friedman’s laboratory, initiated a series of experiments designed to define the nature of the neuronal circuit that triggers feeding-related activity, like biting, in response to sensory stimuli such as hunger or leptin. Kosse identified just such a group of neurons in the mouse hypothalamus, a brain region controlling feeding and other innate drives. Moreover, she devised a way of alternately activating and deactivating the cells in a mouse as it wandered freely about its cage. And that’s when things got strange: As Kosse silenced the neurons, the mouse began to nibble on the metal lick spout of its water bottle. “It looked like something in its brain was directing it to eat the lick spout,” she says.
Indeed, something was. Kosse and her teammates eventually found that by modulating the neurons in question, they could cause their furry subjects to chew on anything that happened to be in front of them, even a block of wood, aptly demonstrating how involuntary the supposedly voluntary act of eating can be. The neurons Kosse identified receive direct inputs from cells that are regulated by leptin and in turn send signals to key brain stem neurons that control chewing and other movements associated with food consumption.
New research is revealing just how involuntary the supposedly voluntary act of eating can be.
The simple feeding circuit Kosse discovered is composed of only three neurons, and its architecture resembles that of an involuntary reflex. Friedman was astonished by the finding, not because he didn’t think that such primitive, reflex-like feeding-related circuits existed (he suspects that many do), but because of the technical challenges involved in mapping one. “What’s shocking to me is that we were able to directly connect inputs to outputs,” he says. Kosse and Friedman believe that simple circuits centered in more primitive brain regions such as the brain stem and hypothalamus evolved early in vertebrate evolution and that their activity is in turn modulated by cortical circuits in mammals that process complex sensory information.
Drawing on the work of two Nobel laureates, the pioneering neurophysiologist Sir Charles Sherrington and the ethologist Nikolaas Tinbergen, Friedman has come to think that feeding and other innate behaviors are controlled by a large ensemble of simple reflex-like circuits. While these circuits are subject to a degree of top-down control, including our conscious efforts to curb our appetites, they are exceedingly hard to resist in the long run. Friedman gravitates to an analogy presented by Sherrington—the urge to cough. A cough is controlled by a simple reflex arc, and it can be stifled for a while but probably not forever, especially if the stimulus (an irritant in the lung) is strong.
The circuit Kosse uncovered is one of the first instances in which a complete circuit connecting inputs to outputs in a mammal has been uncovered. But it is only one in a long chain of discoveries by Friedman and his associates that have defined the biological basis of obesity. Among other things, the discovery of leptin changed the way scientists thought about fat. For example, it allowed Stephen O’Rahilly, a researcher at Cambridge University who found the first genetic causes of obesity in humans, to establish that the congenital inability to produce leptin also resulted in severe obesity in humans. What’s more, O’Rahilly cured his patients nearly instantly by injecting them with the missing hormone.
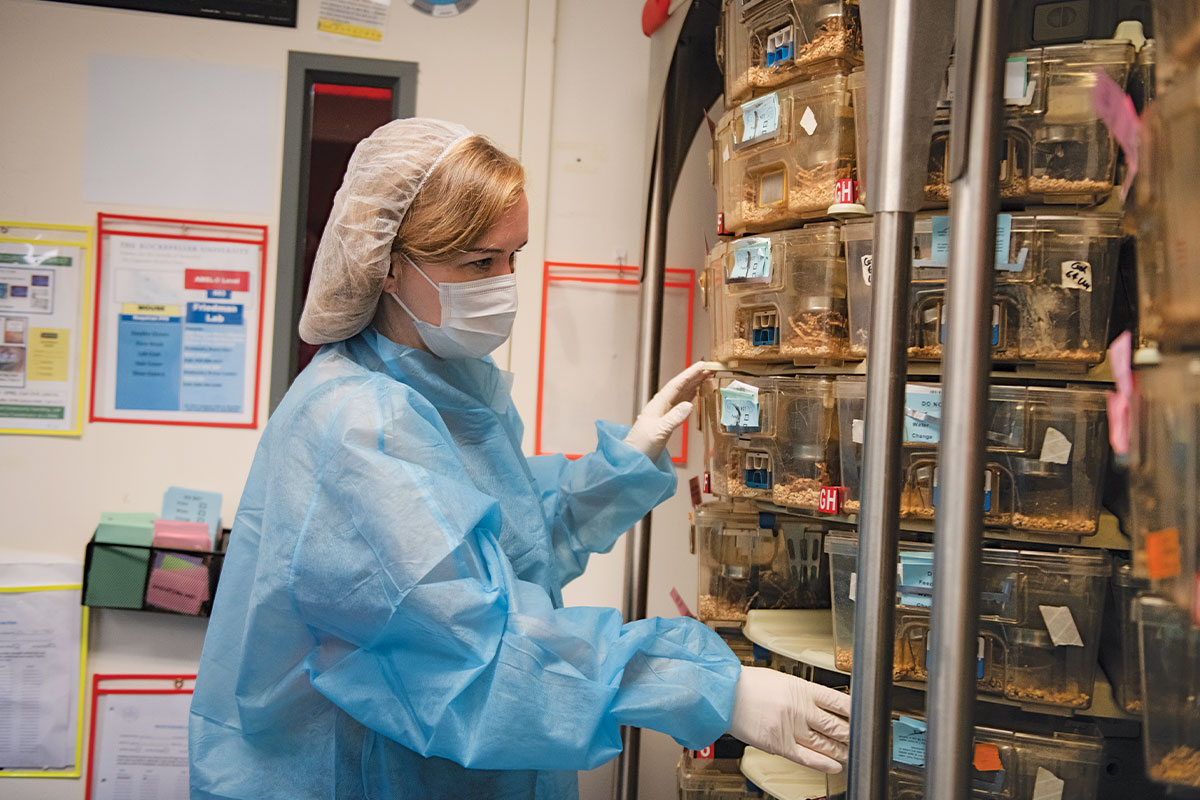
The leptin levels in your blood regulate a neural system that balances the number of calories you consume against the number that you burn over the course of weeks, months, and years. This long-term system for regulating body weight keeps your fat reserves within a narrow range that is largely dictated by your genetic makeup. As you gain fat, you produce more leptin; the hormone signals to the hypothalamus to decrease appetite and increase energy use, causing you to lose weight. Conversely, when you lose fat, you produce less leptin, causing your appetite to increase and your energy expenditure to dip. This is why obese people who shed weight struggle mightily to keep it off: Because their leptin levels fall after weight loss, their bodies are constantly fighting to regain those lost pounds.
Studies have shown that body fat mass is the most heritable trait after height, and scientists have discovered dozens of genes that help regulate body weight as part of the overall system governed by leptin. Mutations in one or more of these single genes cause obesity in humans and in aggregate account for as many as 10–15 percent of cases of morbid obesity (defined as a body mass index, or BMI, greater than 40). Among the remaining population, many other genetic variants are likely to influence whether an individual in the general population is heavy or lean. This, coupled with environmental factors, explains why obesity rates are rising. As high-calorie diets and sedentary lifestyles become increasingly common, more and more people who are genetically predisposed toward obesity end up consuming or retaining the calories necessary to become obese.
People with defective leptin genes, like the ones that O’Rahilly first identified, don’t make the hormone at all. As a consequence, they are ravenously hungry all the time and become morbidly obese even as infants. In fact, some of the children O’Rahilly treated were so heavy that they needed a wheelchair. Giving them leptin, he says, produced nothing short of a miracle, as they shed their excess weight and rose from their confinement.
Science versus stigma
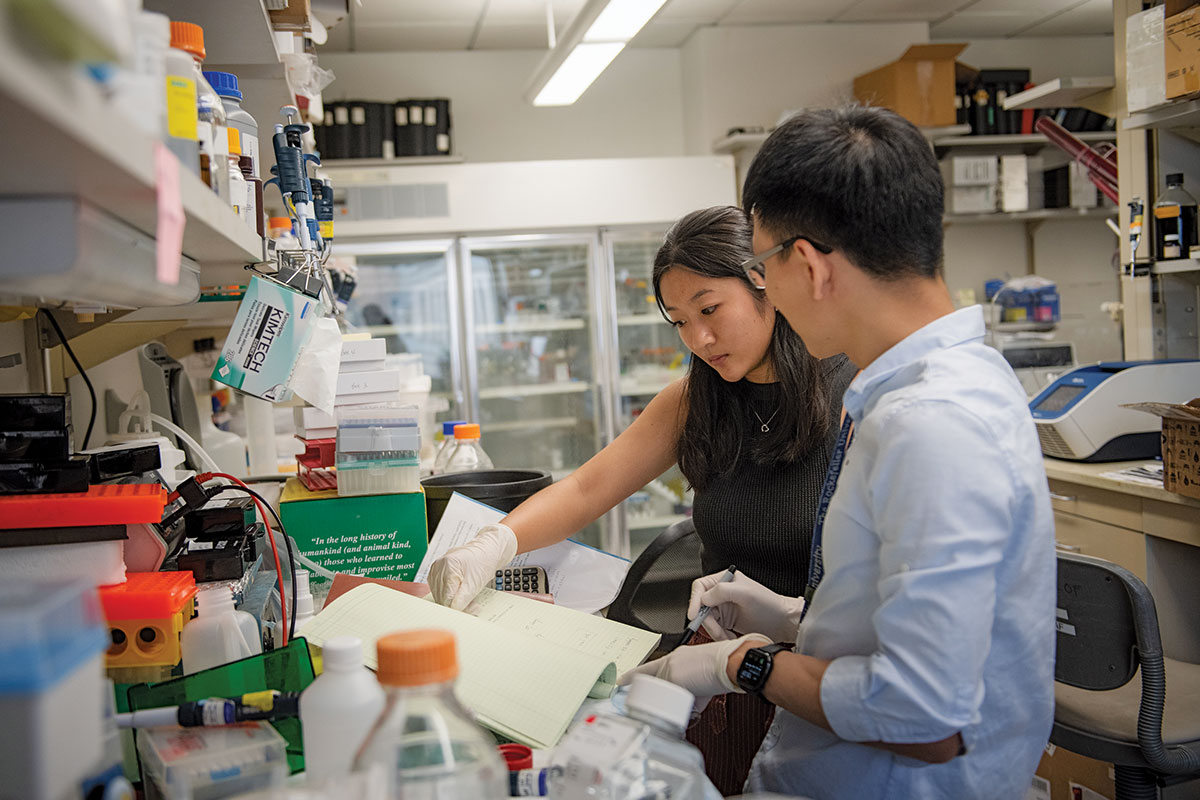
The new generation of weight-loss drugs target specific neurons in the brain stem and hypothalamus that process information conveying feelings of fullness, which can be experienced as satiety or, when extreme, nausea. But even before these new drugs took off, the scientific and medical communities were waking up to a new understanding of obesity. Last year, the American Society of Pediatrics (ASP) called for a new and aggressive approach to curbing the condition in childhood using drugs like Wegovy along with more invasive treatments like bariatric surgery.
This move toward medicalizing obesity at such a young age came as a shock to many, but it was based on alarming evidence. Numerous studies show that obesity has profoundly negative effects on people’s emotional and psychological well-being, often due to the societal stigma they experience (in some cases, these emotional challenges can have as deleterious an impact on health as the weight itself). It can also be devastating to physical health. The nearly 42 percent of American adults who are obese—defined as a BMI of 30 or above (BMI remains the clinical standard, though recently some researchers have challenged its validity)—are more susceptible to ailments including cardiovascular disease, stroke, diabetes, and sleep apnea. They are also more susceptible to ailments including cardiovascular disease, stroke, diabetes, and sleep apnea. Moreover, obesity changes the body structurally, upping the number of fat cells, so that losing weight becomes increasingly difficult.
For children, obesity locks in a lifetime of problems. But for the ASP, the most concerning evidence had to do with the limits of diet and exercise: Study after study has shown that while people can temporarily shed pounds by eating less and moving more, they almost invariably regain them over time, presumably due to the tight grip exerted by leptin over fat mass and metabolism.
By establishing that obesity is seldom remediated by lifestyle choices alone, Friedman’s research offers a revolutionary alternative to fighting it. His findings prove that food intake and metabolism are tightly regulated by a physiological system, reframing excessive weight as a problem of biology rather than willpower, of genes and molecules rather than gluttony and sloth.
And yet the myth of willpower endures, even among health care professionals. “Some of my colleagues in the medical profession tell me, ‘Well, I do still think people should just show more discipline and get themselves together,’” says Matthias Tschöp, a German researcher who discovered a new category of diabetes and weight-loss drugs that combines multiple therapeutic agents in a single medication. These included the approved diabetes and weight-loss drug tirzepatide (sold under the brand names Mounjaro and Zepbound) and other novel drugs that promise to be even more effective than the first breakthrough medications based on the drug semaglutide (sold under the brand names Ozempic, Wegovy, and Rybelsus).
Friedman and his colleagues remain undeterred. By tracing the purely biological pathways that regulate appetite and body weight, they continue to chip away at the idea that obesity can be countered simply by making the right lifestyle choices. Their work not only has opened up fresh avenues to treating and preventing obesity but also has led researchers to rethink the nature of fat itself.
The good grams
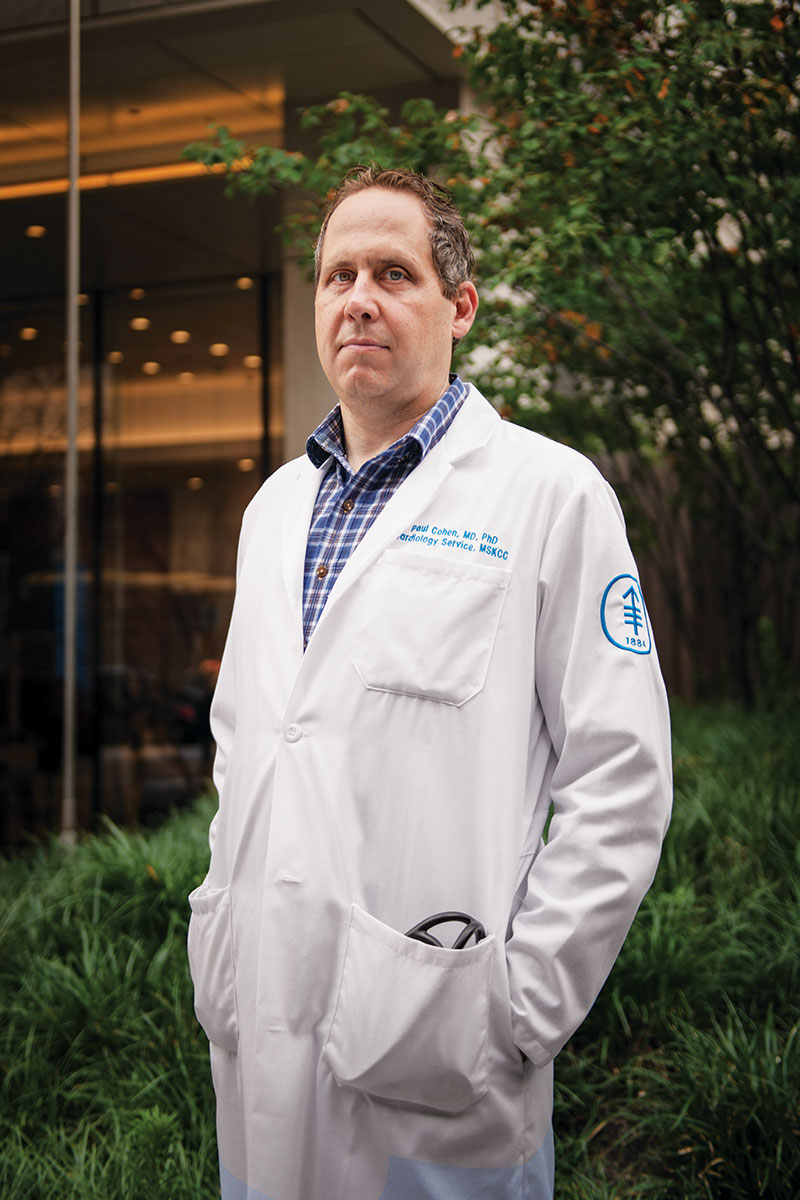
Part of what makes obesity such a complicated disease is that fat isn’t just one thing. In fact, it isn’t even the one thing it was thought to be.
“Many people didn’t accept that fat is a tissue in the same way that the lung or the heart or the liver is a tissue; they just thought of it as a simple bag of cells,” says Paul Cohen, head of Rockefeller’s Weslie R. and William H. Janeway Laboratory of Molecular Metabolism and a former student of Friedman’s. By demonstrating that white fat—scientifically known as adipose tissue and the main storage depot for calories—wasn’t just a sack for storing excess calories but rather a dynamic organ that sent hormones through the blood into the brain, Friedman inspired others to wonder what else it might do.
Cohen is one of them. As a cardiologist who regularly sees patients at Memorial Sloan Kettering Cancer Center (MSK), he is all too aware that cardiovascular disease is a leading cause of death and that obesity is a major risk factor for it. More broadly, obesity is a component of metabolic syndrome, a cluster of conditions that greatly increase the risk of diabetes, stroke, and heart disease. “If you take care of patients,” says Cohen, who is also Rockefeller’s Albert Resnick, M.D. Associate Professor, “you see how common excess body weight and obesity are and how many different health outcomes they influence in patients.”
Precisely how excess fat influences metabolic and cardiovascular health on a cellular and molecular level remains something of a mystery, however. To solve it, Cohen investigates the fundamental biology of adipose tissue itself.
“Many people didn’t accept that fat is a tissue. They just thought of it as a simple bag of cells.”
His clinical work both fuels and informs his research. Some of the patients Cohen sees at MSK, for example, are adult survivors of childhood cancer—a group that tends to develop metabolic disorders such as hypertension and high cholesterol relatively early (i.e., in their 20s and 30s, as opposed to their 50s and 60s) and to die at unusually high rates from cardiovascular disease even when they aren’t obese. Cohen and colleagues at MSK wondered if radiation therapy early in life might have caused these health problems by damaging the patients’ fat tissue, and a study they conducted at The Rockefeller University Hospital revealed that childhood cancer survivors who had been treated with radiation displayed an inflammatory gene signature in their adipose tissue, indicating that their fat cells had in fact been permanently injured.
Cohen’s work as a clinician has also led to groundbreaking work on a special form of adipose tissue known as brown fat.
Unlike the white fat that tends to pool around our bellies and thighs, brown fat collects in far smaller amounts above the diaphragm (some people have modest deposits, while others have almost none). Moreover, it does not produce leptin. Brown fat has other important functions, however: When the temperature plummets, it burns energy to generate heat. And as Cohen demonstrated in a landmark 2021 study, people with more brown fat activity are at lower risk of developing the various illnesses associated with obesity.
That work also emerged directly from Cohen’s clinical experience. More than a decade ago, physicians discovered that brown fat shows up on a particular kind of PET scan that is routinely used to detect cancerous tumors. Soon after he began seeing patients at MSK in 2016, Cohen realized that the hospital must perform thousands of such scans every year. Better yet, he learned that radiologists there routinely recorded the presence of brown fat deposits.
Cohen and his colleagues analyzed over 140,000 PET scans from more than 50,000 patients, linking the presence or absence of brown fat in their bodies to all of the other information in their electronic health records. Patients with detectable brown fat deposits were at lower risk of everything from high cholesterol to heart disease and type 2 diabetes. Somehow, brown fat protected them from a whole host of metabolic and cardiovascular ills. And the more obese they were, the more it helped.
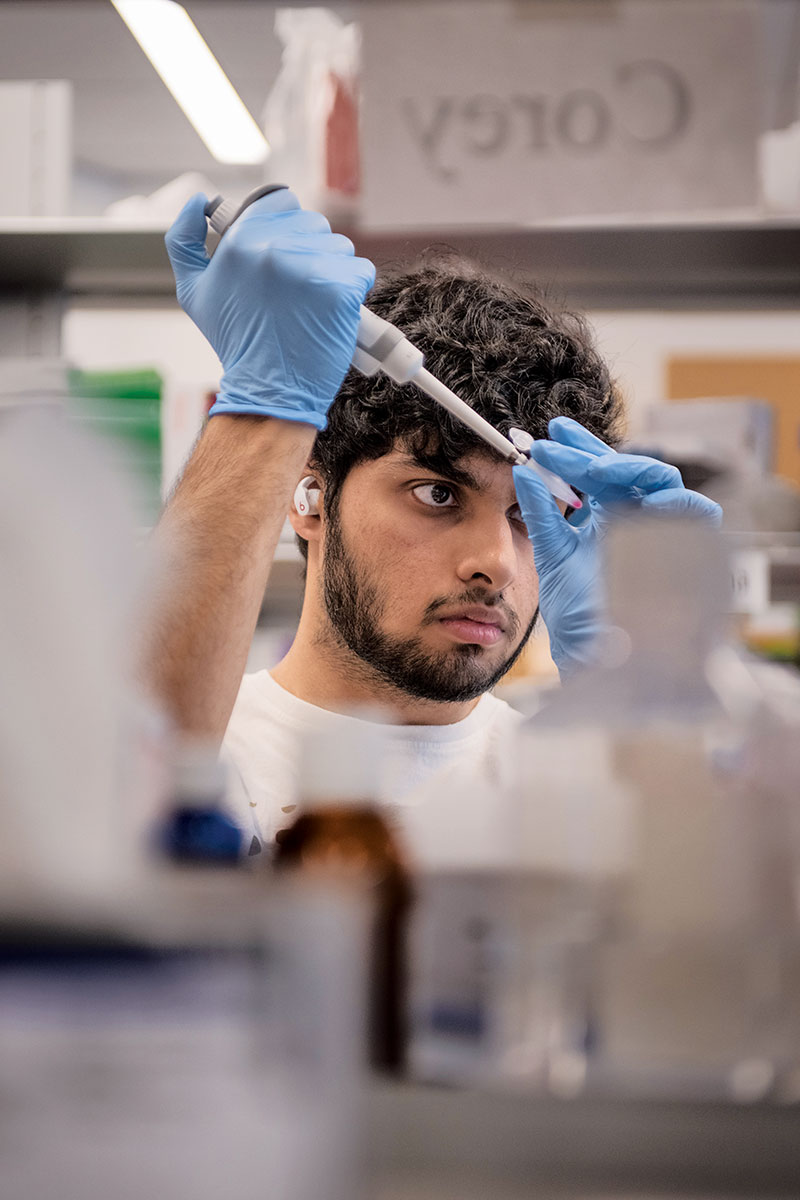
Cohen and his MSK partners are now sifting through the same trove of patient data to determine if particular genetic variants might account for how much brown fat a person has and to see if particular medications can influence brown fat activity. What they learn could eventually help boost the protective effects of brown fat.
At the same time, he and his lab mates are trying to figure out exactly how brown fat confers its various benefits in hopes of replicating them.
Research by Mascha Koenen, a postdoctoral associate in the Cohen lab, suggests that brown fat may prevent the small arteries that regulate blood pressure from stiffening, a finding that could lead to novel therapies for hypertension—yet another constituent of metabolic syndrome and one that contributes to millions of deaths each year from heart disease, heart attack, and stroke. “You don’t die from obesity; you die from the comorbidities,” says Koenen. “So this protection from cardiovascular disease is super important.”
Research associate Kaja Plucinska, meanwhile, is investigating whether brown fat works its metabolic magic by secreting its own unique repertoire of hormones. Toward that end, she is analyzing the blood of people who have been exposed to cold to see what molecules brown fat might be pumping into their bodies. Such molecules could be used to measure brown fat activity, predict a person’s metabolic status, and treat diabetes and cardiovascular disease.
As part of a human study, Plucinska took blood from a small group of young, healthy individuals, had them wear so-called cold vests chilled with circulating cold water, and then took their blood again, screening both sets of samples for thousands of different substances. This past winter, she worked with researchers in Minnesota to collect blood samples from several hundred “ice dippers,” who regularly hop into frozen lakes, and she plans to compare those samples with ones drawn over the summer to see if any potentially useful molecules stand out. “It’s a fishing expedition,” Plucinska says, “hopefully, one that leads to a good catch.”
Through these and other projects, Cohen hopes to identify molecules that could be used to develop drugs that mimic the effects of brown fat. Such drugs could help protect anyone, regardless of how much weight they carry, from metabolic diseases that lead to illness and death.
Overcoming resistance
Friedman’s early work encouraged other scientists to explore the molecular underpinnings of the body’s weight-control system. Tschöp, for instance, recalls thinking that the discovery of leptin represented “the end of obesity.” Inspired by Friedman’s example, he eventually discovered that another hormone, ghrelin, acts as leptin’s nemesis, driving hunger and food consumption. Now history appears to be proving his initial hunch right, albeit at its own measured pace.
As it turns out, only a tiny fraction of obese individuals do not manufacture leptin, while perhaps 10 percent don’t produce quite enough and would benefit from receiving more. The other 90 percent, meanwhile, manufacture plenty of the hormone but don’t respond to it in the way that they should, a condition known as leptin resistance. This resistance renders leptin treatment ineffective for most obese people.
Nonetheless, the discovery of leptin and the astonishing effect it produced in a small number of genetic outliers offered scientists a powerful tool for tracing the biochemical, genetic, and neural pathways that regulate body weight in general, and established a road map for finding ways to prevent and treat obesity in the broader population.
Semaglutide and tirzepatide, for instance, circumvent leptin resistance by targeting a physiological system that regulates food intake over the short term. This system is controlled by hormones and neural signals originating in the gut, and instead of regulating body weight over months and years the way leptin does, it determines how much food you are likely to consume over the course of a single day. Semaglutide creates feelings of satiety by mimicking the gut hormone GLP-1, whose active form was first identified by Rockefeller scientist Svetlana Mojsov. Tirzepatide, meanwhile, mimics both GLP-1 and a second gut hormone called GIP.
Unlike earlier weight-loss drugs, which were ineffective at safe doses and unsafe at effective ones, these new therapies can safely help obese individuals lose enough weight (more than 15 percent of their body weight with semaglutide or more than 20 percent with tirzepatide) to significantly improve their health. They are not for everyone, however. The new drugs are very expensive and carry side effects (e.g., nausea, vomiting, and stomach pain) that some people cannot tolerate. Moreover, they must be injected rather than taken orally (although pharmaceutical companies are working on making them in pill form). And while they are widely effective at decreasing and even normalizing body weight, the genetic complexity of obesity virtually guarantees that they will not work for everybody. Nor, as Tschöp points out, do they actually cure anything: When the treatment stops, the weight comes back. As a result, the need for additional therapies remains acute.
A war on multiple fronts
Since his original discovery, Friedman has been exploring two separate sets of questions. One focuses on intervening in the long-term system for regulating body weight at a point where leptin isn’t directly involved, so that leptin resistance isn’t an issue. The other involves reversing leptin resistance itself.
Several years ago, Friedman and his colleagues identified a group of neurons in the brain stem that, when activated, caused mice to lose weight. While these neurons are connected to the leptin circuitry in the brain, they are nonetheless capable of promoting weight loss even in leptin-resistant and leptin-deficient animals. In research recently posted on bioRxiv, Friedman and his team identified an oral drug that causes weight loss in obese, leptin-resistant mice by targeting these specific cells, a finding that could someday lead to an oral therapy for leptin-resistant human beings.
Furthermore, the neurons that caused Christin Kosse’s mouse to gnaw on a metal lick spout can also regulate feeding behavior in the absence of leptin, suggesting they might potentially serve as targets for novel weight-loss treatments. In addition, there are likely to be additional populations of cells operating other basic feeding circuits that could be targeted in a similar manner by finding drugs that modulate their activity.
But the key objective has been to decipher the cause of leptin resistance. In recent studies, Friedman lab members Kristina Hedbacker, a research associate, and graduate fellow Bowen Tan found a previously unknown source of leptin resistance, as well as a way to overcome it. They initially set out to find biomarkers for leptin sensitivity. In the process, the pair discovered a distinct molecular signature in mice that were fed a high-fat diet known to cause obesity and leptin resistance. The molecules comprising that signature were associated with a specific biochemical pathway centered on a key signaling molecule known as mTOR. So Hedbacker and Tan decided to see if they could induce weight loss in their obese, leptin-resistant mice by giving them leptin in conjunction with rapamycin, an immunosuppressive drug that inhibits mTOR signaling. “By the third day of the experiment, we started to see that the animals were losing weight,” Hedbacker says. “The fourth day, it still held up, and we were so excited that we were screaming.”
Over the next 10 weeks, the obese, leptin-resistant mice that received leptin with rapamycin lost over 20 percent of their body weight and had their responses to exogenous leptin restored. Further investigation pinpointed the specific leptin target neurons and biochemical changes that had caused leptin resistance in the animals, and revealed how rapamycin reversed it.
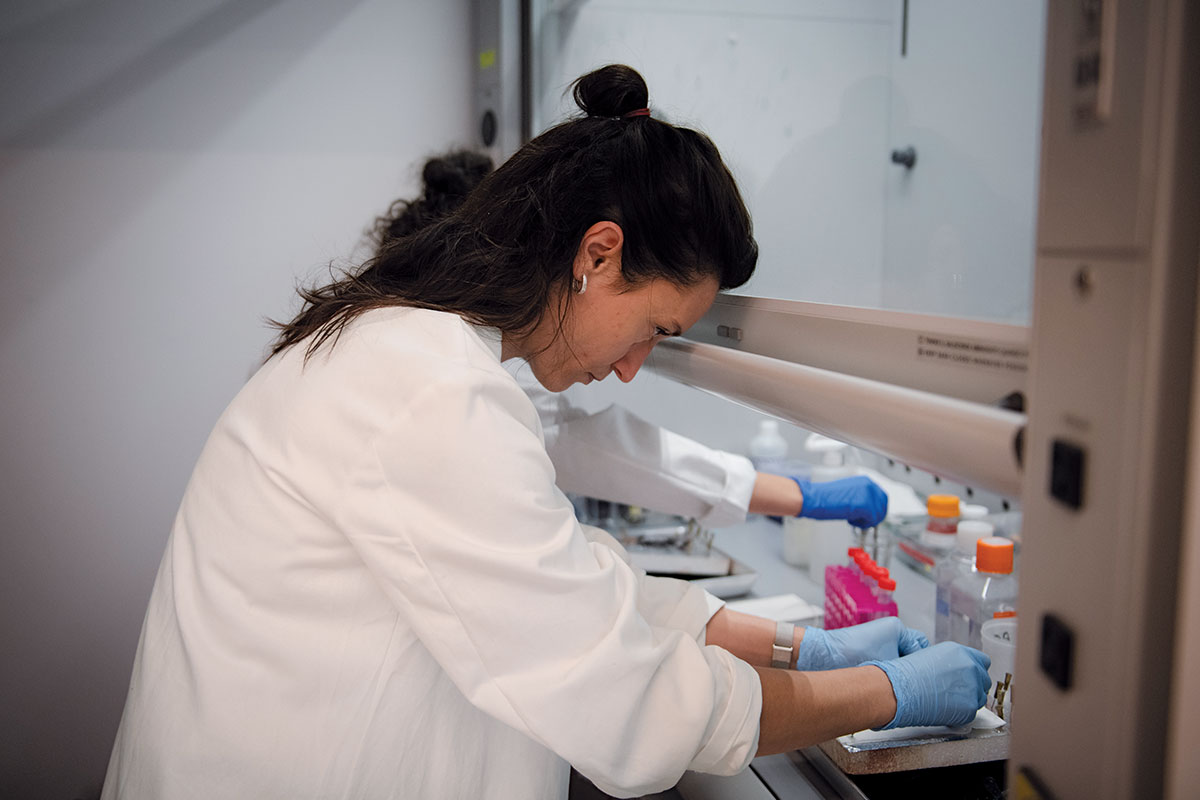
The beginning of the end
These discoveries and others are helping to unravel the neural and molecular factors that shape our feeding behaviors and prevent or promote obesity and the comorbidities that can result from it. They also point to a portfolio of future drugs tailored to patients with different genetic profiles.
Drugs based on gut hormones, like Wegovy and Zepbound, might be combined with leptin for even greater efficacy. People who lose weight with gut-hormone-based drugs might be given a leptin-resistance inhibitor to prevent them from regaining it, while people who can’t tolerate such drugs might be given an entirely different medication that targets a specific feeding-related circuit. And anyone afflicted by the metabolic disorders associated with obesity, whether obese or not, might be prescribed drugs that mimic the effects of brown fat.
There is even reason to hope that as this future unfolds, the stigma associated with obesity will begin to wane, both at an individual level, by helping obese people achieve lasting weight loss, and at a societal one, by making it clear that obesity is not a sign of moral weakness but rather a biological condition amenable to medical intervention.
“There are lots of stigmatized disorders that over time have been destigmatized,” Friedman says. “To some extent, they get destigmatized when there’s a treatment.”
While the end of obesity still lies at some remove, science may have finally brought the beginning of the end into sight.