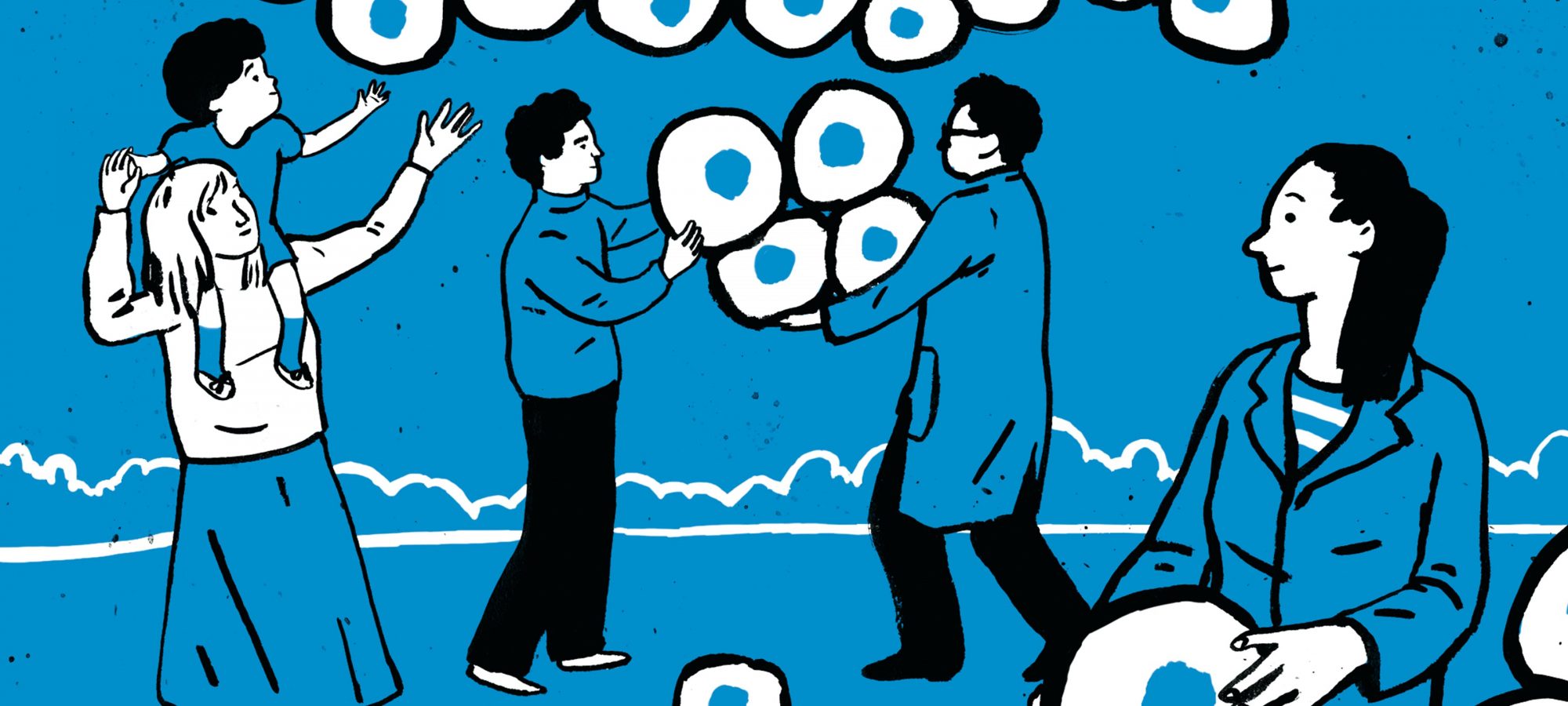
Feature
The 7000 Fields That Science Forgot
Research on rare diseases has more to offer than meets the eye—including the promise of discoveries that could help advance all of medicine.
By Eva KieslerFame equals fortune, even in the world of disease. A small number of well-known disorders get the lion’s share of the attention while more than 7,000 diseases classified as rare or “orphaned” go largely unstudied.
“It’s a real problem,” says Sanford M. Simon, whose lab investigates a group of rare childhood cancers that thus far have received next to no federal research support.
This isn’t to say we should be focusing less on things like breast cancer or Alzheimer’s—clearly, conditions that affect millions are crucial to investigate. Rather, the problem is our failure to simultaneously pay attention to other, more-obscure maladies, many of which have long been overlooked by research funding agencies, pharmaceutical companies, and other systems that medicine relies on to move forward. Lacking the muscular advocacy groups that lobby Congress and organize fun runs, the majority of rare diseases remain understudied, mysterious, and untreatable.
The lack of progress is obviously a problem for the patients Simon works with—or, for that matter, anyone who has been diagnosed with a condition that doctors don’t know how to treat. What may be less obvious is the opportunity at stake for society at large. Patients, it turns out, are not the only ones who stand to benefit from more research into unexplored afflictions.
In fact, experience shows that the information gained by looking under the hood of a rare disease often has implications for science at large. Increasingly, those pursuing this work are finding unique windows through which to observe the maneuverings of genes, cells, and biological systems, empowering them to crack open new fields of investigation. Such off-the-beaten-track discoveries can be surprisingly potent, sometimes advancing medicine in unforeseen ways.
“Rare diseases often represent well-defined biological questions,” says Simon, “and studying them can reveal insights into basic biology, physiology, and pathology, with far-reaching impact. It may be counterintuitive, but I’ve come to believe that furthering our understanding of these conditions would be a great long-term investment for our country.”
Here are three examples that are already paying off.
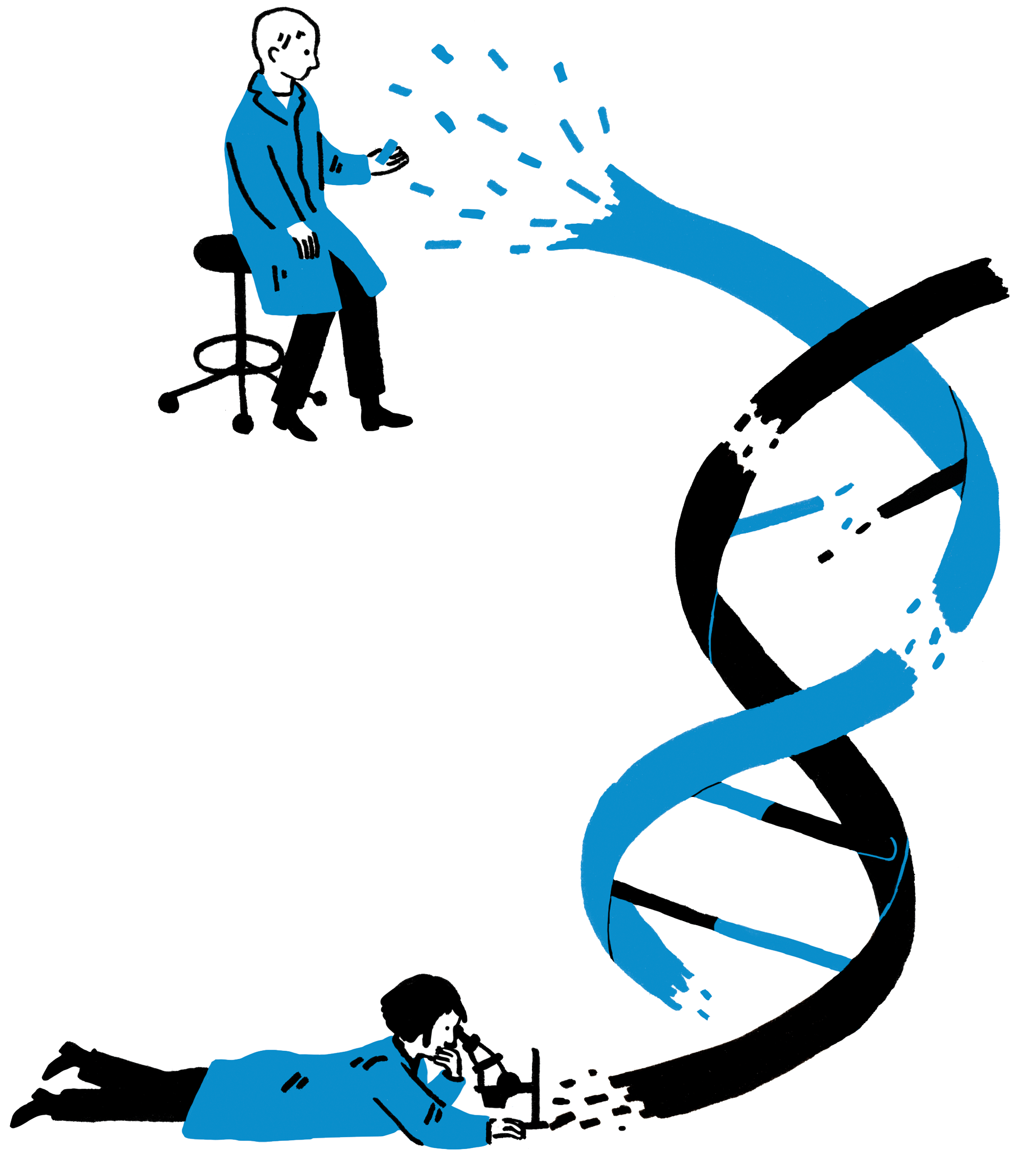
Fanconi anemia
An up-close look at how DNA stays safe
It is sometimes said that diseases are the experiments of nature. Notwithstanding the grim twist of that metaphor (if nature is conducting experiments, we’re all lab rats waiting to be plucked by our tails), it is apt in the sense that some disease-altered cells and tissues provide a useful setup in which to ask certain kinds of biological questions—almost as if they had been designed for that purpose.
Agata Smogorzewska had this realization as a postdoc, working in the lab of Harvard geneticist Stephen Elledge to study the repair mechanisms cells rely on to fix breaks or typos in their DNA. She became captivated by the mysteries of a rare disease almost by accident, after she discovered a previously unknown component of the cell’s DNA-repair tool kit.
‘You think you understand how DNA is repaired. But then you talk with patients about their experience, and your gut tells you that you don’t have the full picture.’
When Smogorzewska depleted cells of this component, a protein called FANCI, and then exposed the cells to a DNA-destroying chemical, they were unable to recover from the insult they way a normal cell would. Under the microscope, their chromosomes looked weird: Some were broken while others sported eccentric shapes.
A pathologist, Smogorzewska happened to know these shapes: They looked like positive results from a clinical test for Fanconi anemia, a heritable disease that often causes bone-marrow failure, developmental defects, and cancer. Of course, the resemblance wasn’t a coincidence. As Smogorzewska soon found, some patients with previously unexplained forms of the disease turned out to have mutations in FANCI—in other words, their disease likely stemmed from the very type of defect she had engineered into her lab cells.
The discovery was a turning point in her career. “The more I looked at the cells,” she says, “the more questions I had—about the disease, about DNA repair, and how the two fit together.”
Over the past 10 years, Smogorzewska, now an associate professor and head of the Laboratory of Genome Maintenance, has established a range of methods to explore DNA repair by using Fanconi anemia as a backdrop. Her lab has now identified several other mutations that can lead to the disease—there are at least 22 of them—and the team is tracing these findings back to the molecular processes that cells employ to keep their DNA intact.
“Understanding the basic biology is really essential,” Smogorzewska says, “and could be helpful in research on several diseases.” For example, she and her colleagues are exploring the role of BRCA2, a protein that is altered in some patients with Fanconi anemia as well as in subsets of women with breast or ovarian cancer. Cells normally rely on this protein to patch up so-called double-strand breaks in a process that involves mending parallel cracks in DNA’s double helix. Deciphering this mechanism is a first step toward understanding why BRCA2 mutations may cause these widespread forms of cancer.
1 in 10 Americans suffer from a rare disease. About half of them are kids.
At the same time, the work remains highly relevant to Fanconi anemia itself. Patients with this disease tend to die young, and while stem cell transplants are often effective for bone marrow dysfunction, the disease causes many other problems, including head and neck squamous cell carcinoma, a relatively common type of cancer. The lab maintains a registry with clinical information and samples from over 1,000 patients and their family members; Smogorzewska is using that data to begin to parse out why individual patients are prone to developing particular tumors, hoping to eventually discover ways to prevent or treat these cancers.
The work requires the lab to constantly go back and forth between lab experiments and the real thing: patients and the disease they live with.
“Based on lab experiments alone, you think you understand how DNA is repaired,” Smogorzewska says. “You’ve identified the molecular players in a process, you think you’ve figured out what they do. But then you talk with patients about their experience, and your gut tells you that you don’t have the full picture. Something’s lurking that you don’t understand, which raises new questions—and you’re back in the lab for more experiments.”
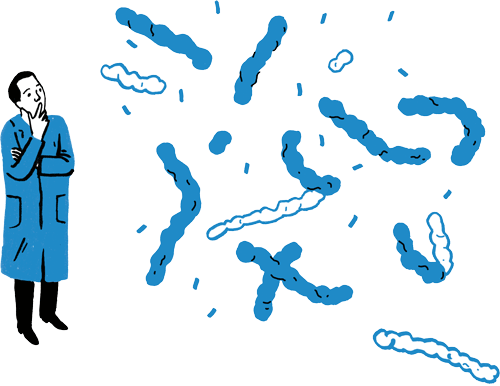
TYK2 tuberculosis
A rare disease that’s actually quite common
As many scientists will tell you, there’s something dubious about calling a disease “rare” or “common.” Some will go even further.
“Diseases don’t exist,” says Jean-Laurent Casanova, a geneticist who studies how certain mutations affect people’s vulnerability to infections.
That is to say, diseases don’t exist in the way other biological entities—genes, cells, people, and so on—exist. They make useful headlines but will invariably crumble under scrutiny, dissolving into subtypes and any number of sub-subtypes. “When we use a word like diabetes,” Casanova says, “we are really talking about a vast group of biological derailments. In reality, each patient has a unique form of the disease.”
80% of rare diseases have been traced to DNA mutations.
8 years is the average time it takes for a person with a rare disease to obtain a correct diagnosis.
This is true even for diseases in which an external pathogen, such as a bacteria or virus, is the culprit. Casanova, now head of the St. Giles Laboratory of Human Genetics of Infectious Diseases at Rockefeller, discovered this himself in the mid-1990s, while finishing his pediatrics training in a Paris hospital. At one point, he learned about odd cases of children becoming inexplicably ill after receiving a standard tuberculosis vaccine previously proven safe and effective. Doctors couldn’t explain why the vaccine, which contained disseminated BCG bacteria believed to be harmless, would suddenly induce life-threatening symptoms in these exceptional kids.
Determined to get to the bottom of this mystery, Casanova embarked on an exhaustive study into the pathology of one patient whose reaction to the vaccine had been particularly dramatic. It took him six years, but he solved it: A rare mutation had left a blind spot in this child’s immune system, making it unable to cope with the normally benign BCG.
It was the first of many similar discoveries that Casanova has made since. Over the past two decades, his lab has developed increasingly advanced genomic approaches to explore the relationship between our genes and our immune system, and has used these methods to uncover hidden genetic vulnerabilities to numerous infections, from herpes simplex to influenza, pneumonia, and brain encephalitis. While many of these alterations would previously go unnoticed until the pathogen attacked—at which point the patient’s health might deteriorate quickly—they can now be detected ahead of time, making the conditions more manageable.
‘When we use a word like diabetes we are really talking about a vast group of biological derailments.’
For instance, Casanova’s team recently reported that many people carry mutations in the gene TYK2 that severely heighten their risk of developing tuberculosis. The alteration is particularly common in Europe, where about one in 600 people have it—although, as Casanova points out, most people will never know they are carriers since the risk of infection is low in most European countries. Nevertheless, TYK2-associated TB may be a ticking bomb among globe-trotters. Imagine, for example, that you’re a British health care worker inspired to volunteer in a refugee camp in Myanmar, where TB is rampant. Before entering that environment, you may want to make sure you don’t have the TYK2 mutation, which would render such an expedition very dangerous. “Now that we’ve discovered the mutation,” says Casanova, “people will be able to take a genetic test before traveling to assess their risk.”
Moreover, in exploring the mechanism by which the gene normally helps the immune system function, he and his colleagues were able to identify an existing medicine that, theoretically, might be useful in treating the disease in those already infected. (Read more about tuberculosis in “TB is changing.”)
While it’s easy to appreciate the broad impact of Casanova’s current work, it is also easy to forget how it began—with an in-depth exploration of a single, unusual case. All of which highlights a crucial fact about medical outliers: Their significance in relation to other people’s health will often go unnoticed—unless, of course, someone decides to take a closer look.
Fibrolamellar carcinoma
Building knowledge and community
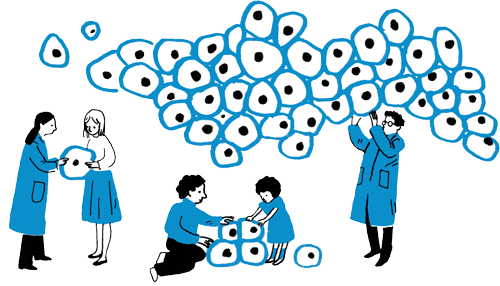
As would be the case for any parent in his situation, Sanford M. Simon’s world fell apart in 2008 when he learned that his 12-year-old daughter Elana had fibrolamellar hepatocellular carcinoma (FLC), a rare and usually lethal form of liver cancer. But unlike most parents with this experience, Simon was able to channel his fear and frustration into his work. A Rockefeller biophysicist, he taught himself cancer biology and launched a crusade against his daughter’s disease and other rare childhood cancers.
Despite a bleak prognosis, Elana’s treatment was successful. She grew up, went to college, and remains cancer-free. But most patients with FLC do not survive, and Simon’s work in this area feels as urgent as ever. If federal research funding is insufficient for rare cancers in general, it is virtually nonexistent for a subset of rare cancers affecting children and young adults. “These are the hardest cancers to raise money for,” says Simon, “yet they are really worth going after. It’s easier to learn new things about cancer if you study it in young people.”
95% of rare diseases lack an FDA-approved treatment.
1 in 2 rare diseases do not have a foundation or advocacy group to promote research and help patients.
This is because, from a genetic point of view, adult tumors are full of noise. They contain lots of mutations, most of which are not responsible for fueling the cancer but have arisen either as a side effect of the cancer or independently of it. Young people’s tumors have not had as much time to acquire mutations, making it easier for scientists to identify the “drivers”—the important mutations that can provide clues about how cancer cells dodge therapy, hide from the immune system, or metastasize in the body, for example. In that sense, rare childhood tumors are low-hanging fruit for biologists seeking to develop better tools for treating any type of cancer.
Recently, Simon and his colleagues—including his daughter, who worked in his lab during high school—identified a single mutation present in each of hundreds of FLC tumors the lab has analyzed. They were able to confirm that this mutation is a bona fide disease driver (“when we engineer it onto mice, it mimics the disease,” Simon says), and they’ve even figured out how it arises: Essentially, a stretch of a chromosome is deleted, causing two genes to fuse together. The result is a faulty gene that produces a cancer-promoting enzyme.
‘These are the hardest cancers to raise money for, yet they are really worth going after. It’s easier to learn new things about cancer if you study it in young people.’
“Here’s a cancer where, only a few years ago, we didn’t even know if it was one disease or many diseases lumped together,” says Simon, who is head of the Laboratory of Cellular Biophysics. “And now we know exactly what the driver is and how it works, and we’re beginning to design therapeutics.”
To that end, the researchers are exploring a novel therapeutic strategy that teaches cells how to recognize and destroy the faulty enzyme. If successful, the same tactic could be applied against several other childhood tumors known to arise from gene fusions—including Ewing’s sarcoma and rhabdomyosarcoma, among others—that together affect tens of thousands of kids.
In addition to the hardship of funding, researchers who study rare diseases are grappling with the challenge of amassing enough clinical material to be able to obtain reliable data. After Elana was diagnosed, her mother, Rachael Migler, established a nonprofit medical registry connecting FLC patients and researchers working on the disease—a network that now includes detailed medical records from more than 150 people all over the world. Thus far, patients have provided Simon and his colleagues with more than 115 tumor specimens, an unusually robust collection for a cancer affecting less than one in five million people. (A few patients who donated tissues have even joined the lab to train as scientists and help advance knowledge about their own disease.)
In turn, members of the lab contribute to the registry by sharing information about rare pediatric cancers, writing easy-to-comprehend updates on the latest research, and answering patients’ questions. “It’s a way to give back to the patient community without which our work would not be possible,” Simon says.