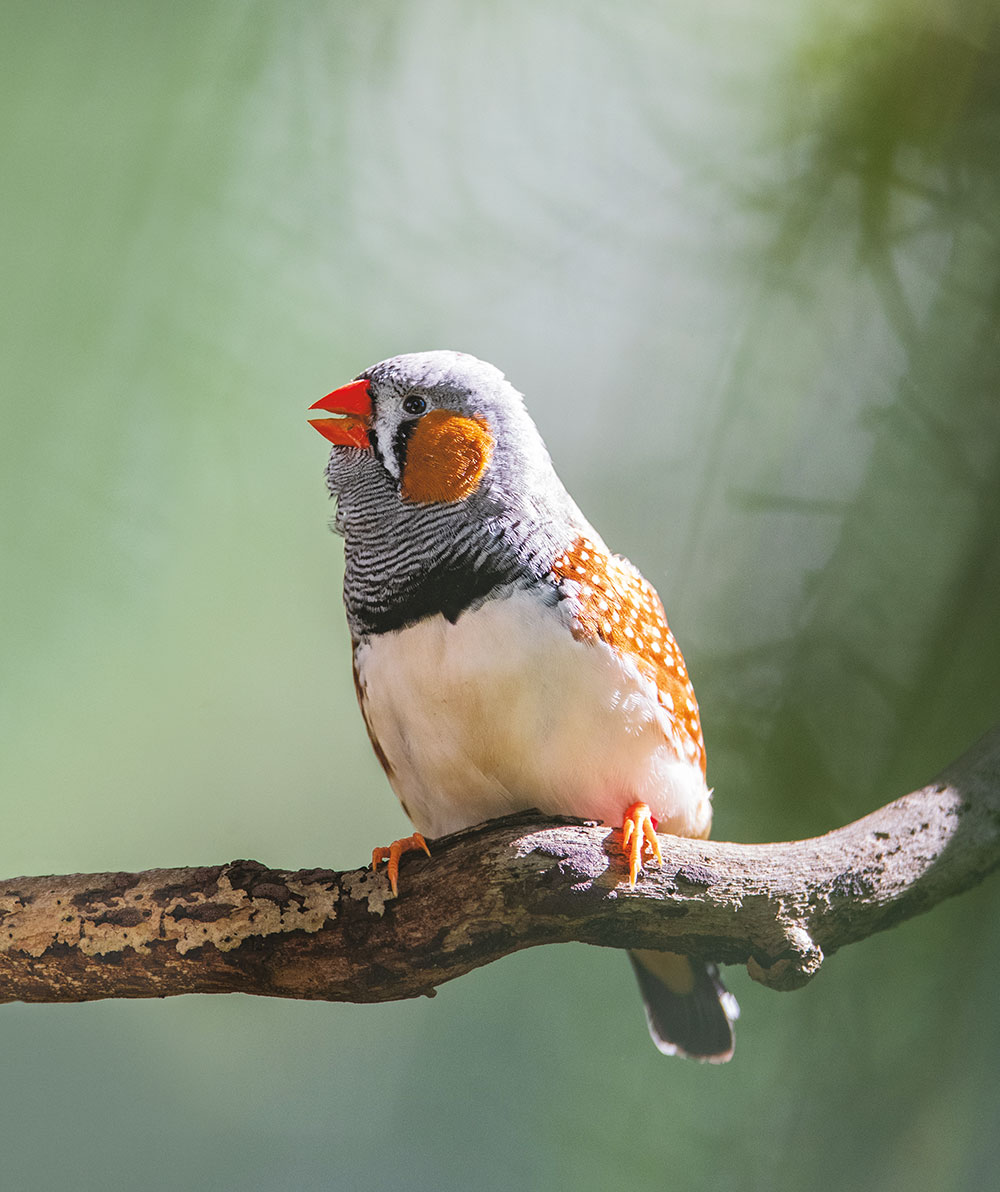
Feature
Repeat after me
How did evolution come up with that most human of all human traits—our ability to imitate and produce speech? The answer has eluded scientists for centuries. But all along it may have lurked above their heads, in the treetops.
By Bahar Gholipour & Eva KieslerHumans are extraordinary. “Paragon of animals,” as Hamlet put it, with “god-like intellect,” Charles Darwin added. We will never run out of words to marvel at our own uniqueness. And we have modern medicine and atomic bombs to show for it.
But while testaments to our specialness abound, it’s been harder to pinpoint what exactly makes us special. Is there something about the human brain that lifts us above our fellow vertebrates? So far, biologists have found no gene, no neural circuit, no anatomical structure that makes us meaningfully different from all other creatures. And one by one, new studies find that other animals possess traits once believed to be quintessentially human: tool use, culture, morality. Even our aptitude for spoken language has turned out to be not so unique.
“Humans are actually one of several animals capable of vocal learning,” says neuroscientist Erich D. Jarvis, his words intermingling with the chirps and squeaks from a nearby cage.
New insights challenge our very notion of what language is, and how it came to be.
As Jarvis continues to talk, he reaches inside the cage and gently retrieves the little noisemaker, a zebra finch small enough to nestle in the palm of his hand. Its cheeks are bright orange, a sign that it’s a male. Jarvis gently deposits the bird into another cage, where two female finches huddle on a perch. The male flutters up to a nearby perch and resumes the concert, and Jarvis explains that the finch’s song is a language comparable to human speech, passed on in its family for generations. Like other songbirds, the finch is capable of vocal learning, a component of language that allows newborn birds to learn to produce sounds with distinct meanings, the closest thing to words and sentences that we know of. It’s a trait that songbirds share with humans and a few other animals—a seemingly arbitrary group that includes whales, bats, elephants, and parrots, among others.
Jarvis likes songbirds (who doesn’t?), but he is not studying them for their own sake. With bird experiments and powerful genomics tools, he uses the finch as a window into the neural constituents of vocal learning, and as a lens through which to illuminate more than 60 million years of evolution. Bit by bit, his lab is reconstructing the trajectories in which birds, humans, and other vocal learners evolved their knack for vocal communication. Their work has already turned up surprising insights, some challenging our very notion of what language is and how it came to be. And ultimately, what the scientists are learning from birds might reshape our understanding of how the human brain produces not just speech, but any kind of behavior, emotion, or thought.
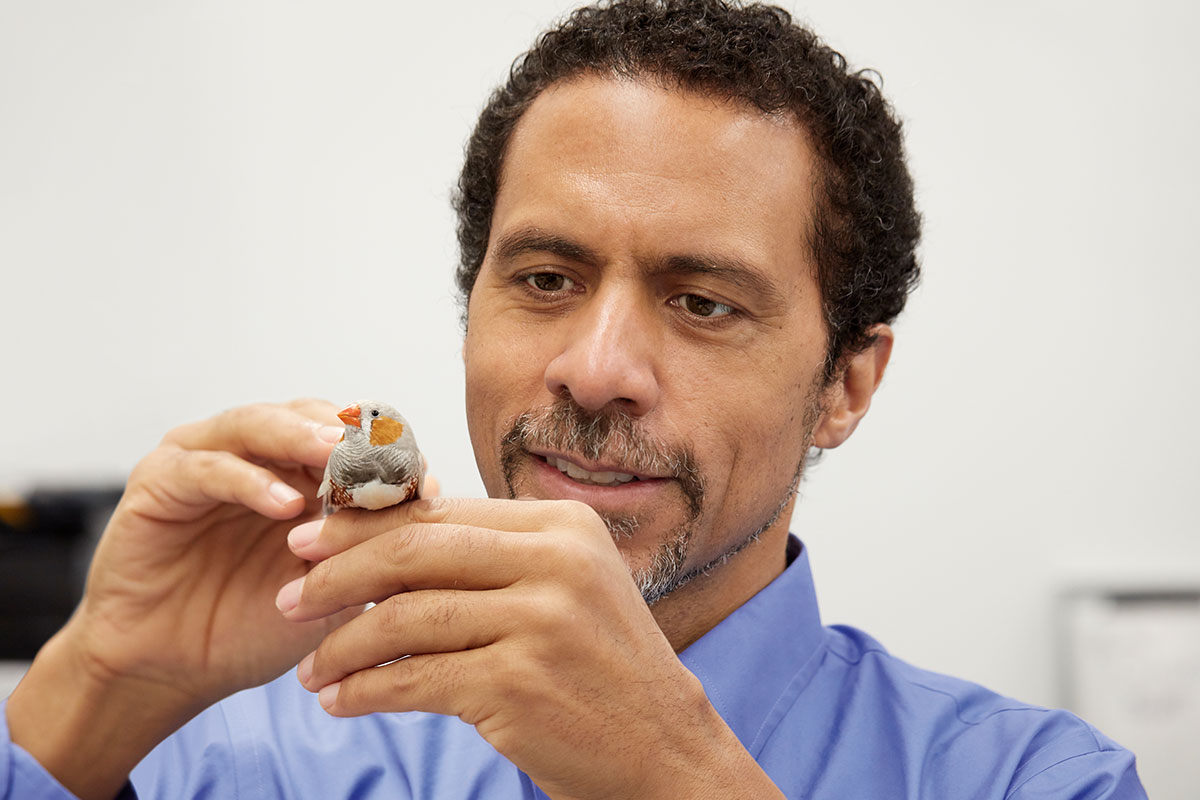
“The most complex brain function we know of is that which enables spoken language,” Jarvis says. “If we can figure out how it works, maybe everything else will fall into place.”
Language is the bedrock of human culture. Without it, our ideas would mostly stay inside our heads, and all the knowledge that’s been passed from one generation to another would be long lost. Whether we’d even be able to think without language is an open question, and there would certainly be no poetry, no stories, no politics, and no science.
But what’s the essence of language, and where did it come from? These questions have been pondered by some of the world’s most notable thinkers, from John Locke to Noam Chomsky, but no clear answers have materialized.
Here’s another question, which Jarvis and his lab members like to chew on: Why us?
“Among the vocal learning species, humans appear to be the most advanced,” he says. It’s tempting to conclude this is because we’re smarter than other animals—until you consider who’s in second place.
“Evolution invented vocal learning several times independently, just like wings evolved separately in insects, bats, and birds.”
But first, let’s consider that while vocal learning is rare, it is just one of several components of language—and some of these other components are widespread in the animal kingdom. “For example, many animals are capable of auditory learning, the trait that allows dogs to learn the meaning of sounds like ‘sit,’” Jarvis says. “Dogs are also capable of vocal-usage learning, the ability to produce a specific sound in a given context, like whining to beg for food.”
So, if humans are the gold medalists in vocal learning, who takes silver? Not the chimpanzee, nor the bonobo, nor the gorilla. Our closest relatives are poor speakers, even though they can be excellent auditory learners (case in point: Koko, a famous gorilla, learned thousands of words but used only sign language to communicate them). The fiercely intelligent dolphin? Closer. Dolphins are indeed vocal learners, but they’re not in the top tier. Second best after humans is none other than the parrot, with the songbird coming in third.
Humans, parrots, songbirds: It’s a strange club. Our ancestors parted ways with the ancestors of birds more than 300 million years ago. How can it be that, of all things, our strongest competitors in vocal learning—the trait that’s supposed to be our edge as a species—are a bunch of feathery dinosaur descendants with brains no bigger than a grape?
For one thing, vocal learning has little to do with the size of an animal’s brain. What matters is the brain’s anatomy. Fernando Nottebohm, a Rockefeller professor emeritus and Jarvis’s former Ph.D. adviser, discovered in the 1970s that vocal-learner birds have specialized brain regions he called song nuclei, whose sole function is to control the animals’ ability to learn new sounds and produce them. These regions are missing in nonspeaking birds, like woodpeckers and chickens.
As a grad student, Jarvis discovered that when songbirds sing, the firing of neurons in their song nuclei causes certain genes to switch on—and for each burst of song, the expression of these genes increases. At the time, in the late 1990s, these findings challenged scientists’ understanding of how learning and memory happens. “When the birds learn a song,” Jarvis says, “this doesn’t just involve changes in synapses, as once thought, but also changes in the neurons themselves.”
Years later, as an associate professor at Duke University, Jarvis was able to expand on these findings and ask at what point a handful of birds evolved song nuclei in the first place. He co-led an international consortium of scientists that spent several years sequencing and analyzing the genomes of 48 contemporary bird species—finches and other vocal learners, and their nearest relatives that are not vocal learners. The result was a stack of 20 papers that, when published near-simultaneously in 2014, landed with a thud in the bioscience world. It gave researchers worldwide a wealth of new data to work on, including a new evolutionary family tree of bird species going back to the extinction of dinosaurs, when most modern birds arose. This exhaustive map upended much of what people thought they knew about birds (it turned out that grebes are more closely related to flamingos than to ducks, for example, and that swifts are closer to hummingbirds than to swallows), and for Jarvis, it confirmed what he had long suspected: that the three species of vocal-learner birds—songbirds, hummingbirds, and parrots—are evolutionarily distant. Each must have acquired song nuclei on its own.
“Over the past 66 million years, evolution invented vocal learning at least three times independently among birds,” Jarvis says, “just like wings evolved separately in insects, bats, and birds.”
Sketched in a diagram, song nuclei make the bird brain look like a flow chart with arrows crisscrossing among seven clouds, each representing a vocal brain region. The arrows trace two neural pathways, one for learning new sounds and another for producing them. When Jarvis showed that this complex neural architecture is practically a mirror image of the human brain’s vocal pathways, some took his findings as evidence for the existence of God. How else, despite being separated by eons of evolution, could two species have been recently upgraded with new brain modules of the same design? Jarvis was perplexed, too, until he stumbled on a major clue in the process of studying birds hopping on an exercise wheel.
Birds are bundles of energy. During experiments, they will not only sing but also walk, sprint, and wing-whir. Jarvis and his colleagues monitor the birds’ brains during these activities either by recording the firing of neurons using tiny electrodes, or by measuring the expression levels of genes known to be sensitive to increased neuronal activity.
This was how the team serendipitously discovered the bird brain’s motor-control regions, which happened to sit right next to the song nuclei. Further experiments showed that in vocal learners, the song nuclei are deeply enmeshed with the motor regions, and they extend neural connections from the cortex all the way down to the neurons in the brain stem controlling the voice box. This could mean that the same pathways that enable chicks to learn to hop or fly also enable them to learn new sounds—a radical finding that other scientists later reported from studies of the human brain.
The upshot is a new way to think about speech: as a motor skill, not unlike riding a bike.
“We think the vocal pathway evolved out of the motor pathway,” Jarvis says, adding that in embryos, motor pathways may duplicate multiple times to connect with various muscle groups. He believes that at one point in evolution, an extra duplication occurred in humans that yielded the specialized motor pathway that controls our voice box—and that such super-duplications also produced the vocal pathways of songbirds, parrots, and hummingbirds.
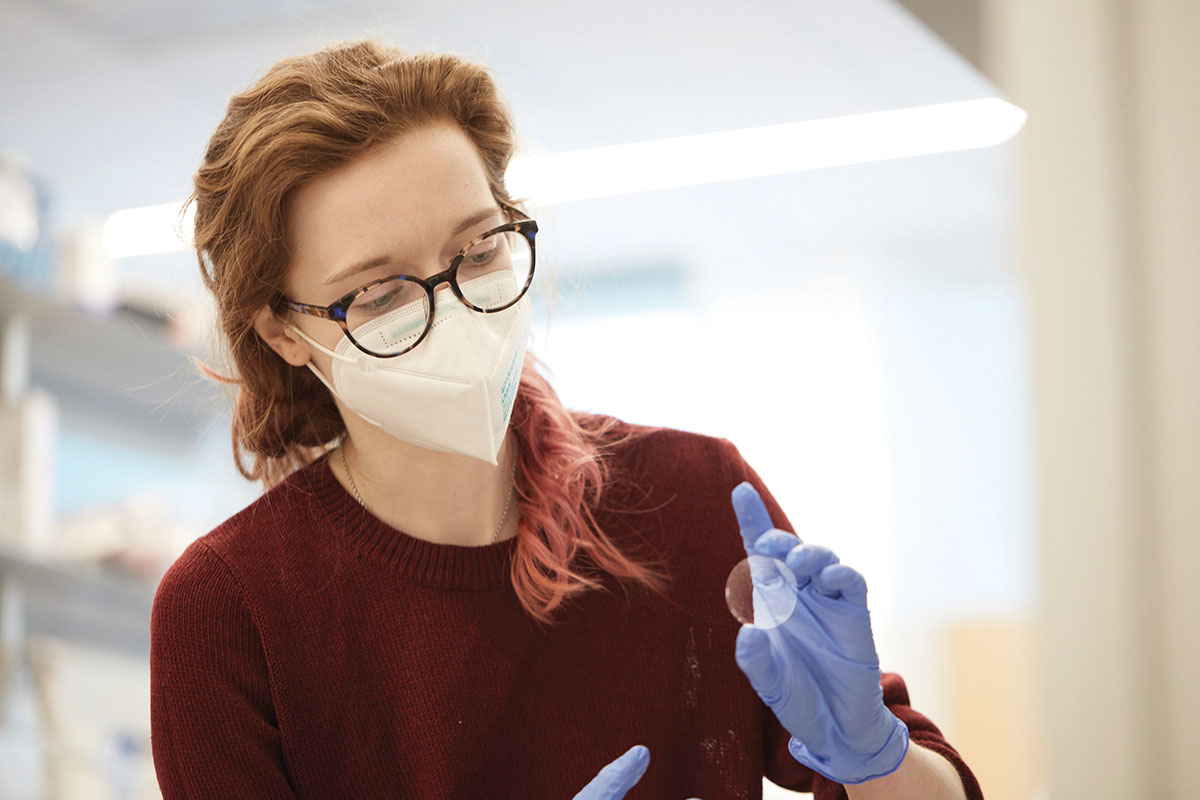
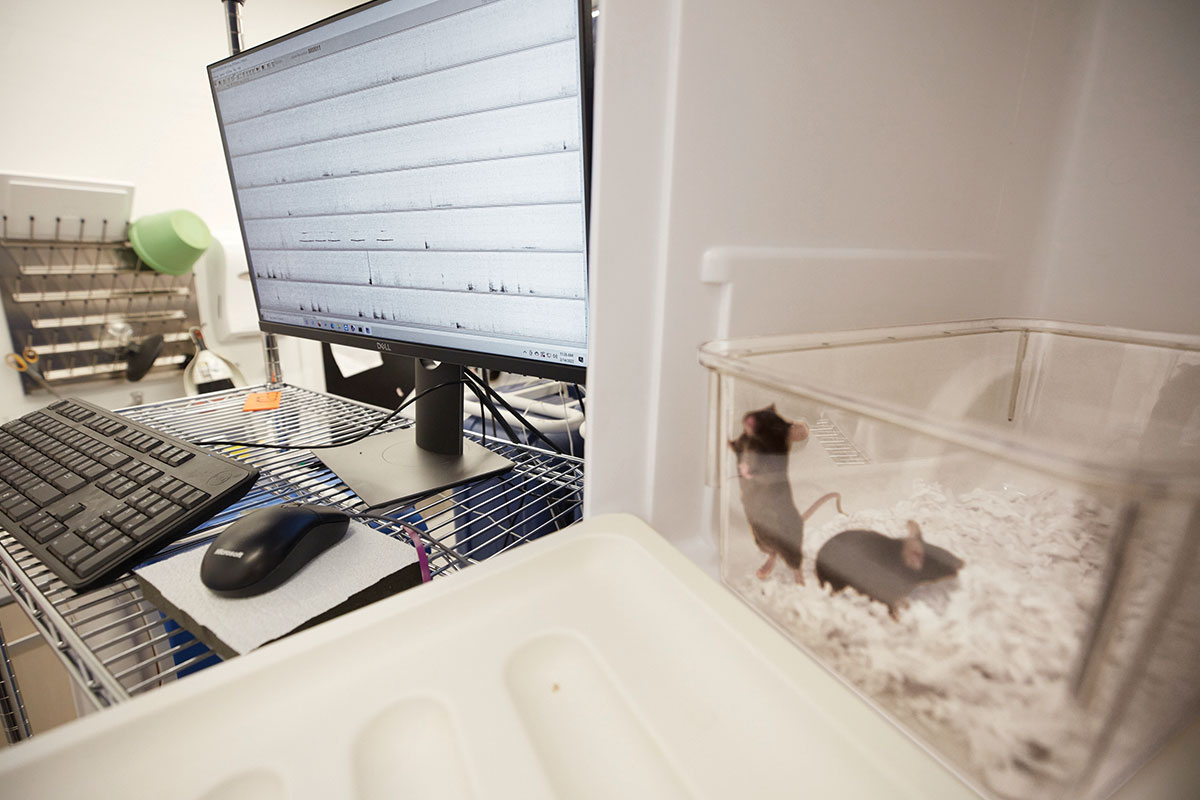
All of which begs the question: If evolution executed this process at least four times, might it also have made other animals like dolphins and bats capable of vocal learning?
Jarvis is not sure, but his team has found a way to peek inside the dolphin brain. When dead dolphins wash up on the shores of Jones Beach State Park on Long Island, graduate student Brigid Maloney hurries over to collect brain tissue. Her goal is to compare the anatomies of vocal pathways of different speaking animals.
“We know that songbirds and humans share a set of genes only expressed in vocal areas,” Maloney says. “We’re trying to see if the same genetic program exists in dolphins.” She has developed a meticulous technique for preparing thin slices from the big dolphin brain, and jerry-rigged a microscope to examine these samples. Her study could illuminate the neurobiology of language in a vocal learner that’s neither human nor bird. (Dolphins and other cetaceans such as orcas are rarely used in neuroscientific experiments, nor are whales or elephants. For starters, they don’t fit in a lab.) And if the researchers’ theory of the motor origin of language is correct, Maloney should trace the dolphin’s vocal pathway to the brain’s motor areas.
Maloney and Jarvis are hoping to find out soon. “Give us a year or two, and we might have the answer,” Jarvis says.
In the meantime, other members of the lab are making headway toward deciphering the genetic underpinnings of vocal learning. Former postdoc Lomax Boyd and graduate student César Vargas have been studying how neurons forming the forebrain-larynx connection regulate a group of genes that are similar across human and vocal-learning bird species, enabling us to learn new sounds from our elders.
Here’s how they think it works: When a neuron expresses a gene named SLIT1, it will not connect with other neurons expressing a receptor called ROBO1 (this is a standard way for developing neurons to know which other cells to connect with and which cells to avoid). In animals that don’t speak, an active SLIT1 gene prevents new pathways from forming between the cortical neurons and brain-stem vocal neurons, which control the larynx (or the bird version of the larynx, called the syrinx). However, Boyd, Vargas, and their colleagues propose that in the speaking species, SLIT1 is silent in the vocal areas. “When SLIT1 is off, the neurons can stay in touch,” Vargas explains. “And that means the animal’s brain can develop fine control over the laryngeal muscles.”
A similar mechanism might exist in humans, although we have an additional layer of control over the formation of neural connections, controlled by a gene called SRGAP2. The result, Jarvis says, is that our brain networks don’t become rigid and unable to continue learning once we grow up, as is the case with adult birds. The multiple layers of fine-tuning keep our brains in a plastic condition, while the brains of other animals become more “solidified,” he says. “The system causes our neurons to stay in a more childlike state, allowing us to learn new vocalizations throughout our lives.”
The genetic mechanisms that keep vocal neurons youthful might be a key additional ingredient of vocal learning, perhaps the very thing that once allowed human ancestors to turn innate grunts into meaningful, complex expressions. Jarvis and his team are now working to test this theory. Would it be possible to reverse engineer the development of vocal pathways and bestow the gift of speech on a nonspeaking animal like, say, a mouse?
Until recently, scientists thought mice completely lacked vocal learning, but Jarvis’s team has found otherwise. During courtship, male mice produce ultrasonic vocalizations that are not unlike birdsong, except for the fact that human ears can’t hear them. The researchers discovered that the animals can sometimes make small modifications to these sounds. When housed in a socially competitive environment, for instance, different strains of mice with different songs will try to match each other’s pitch.
Looking closely at these animals’ brains, Jarvis and his coworkers also found a pale trace of the vocal anatomy of songbirds and humans. “Mice were thought not to have any neuroconnectivity from the cortex to the larynx motor neurons at all,” he says. “But we found a rudimentary connection consisting of very sparse axons.”
The researchers suspect these weak links could be enhanced by manipulating speech genes—for instance, by turning off SLIT1 in the vocal regions of the mouse brain, or by replacing the gene with the human version. If either approach works, the resulting mouse will presumably become more capable of speech-like communication.
A speaking mouse would be more than a scientific gimmick; it would provide an invaluable model for further studying speech as well as speech-related disorders such as stuttering. Because no such model currently exists, a speaking mouse could galvanize research in this area and make it possible to develop new drugs or other interventions.
“It would also give us the first model in which to study the neuroscience of vocal plasticity, or the advanced type of vocal learning that only humans and a few other mammals are capable of,” Jarvis says. “This may well be the most exciting thing we’ve ever worked on.”