Feature
One cell in 10,000
Most cancer cells can’t spread. Find those that can, and the disease could be a lot less deadly.
By Joshua KrischCancer cells aren’t good citizens—they’re selfish and lazy, loitering around in clusters, stealing resources from neighboring cells, and refusing to contribute to the good of the community. But the vast majority aren’t murderers. Out of every 10,000 cells that make up a tumor, 9,999 are basically innocent. Deadbeats, sure, but not criminals.
That one, though? It’s capable of real mayhem.
It can evade the surgeon’s scalpel. Irradiation can’t touch it, and traditional chemotherapies have no effect on it. It’s the cell that breaks away from the initial tumor, setting off on a dogged mission to establish new colonies in critical locations, eventually spreading far and wide. Even when cancer goes into remission, it lives on as a seedling, sprouting months or years later—bringing the disease back with a vengeance. It’s the one cell that’s capable of metastasis.
It’s not a single alteration that makes a cell capable of the varied tasks required to metastasize. It’s more like a perfect storm of them that accumulate in exactly the right way.
Metastasis is the reason cancer is so dangerous. But if you can stop that single cell, you can basically beat cancer, reasons Sohail Tavazoie. Trained as an oncologist and now running a busy lab devoted to the study of cancer biology, Tavazoie’s premise is simple: If we can figure out why a tiny minority of cancer cells have the ability to trigger metastasis, and devise a way to stop them, cancer becomes a lot less scary.
It was the mid-2000s when Tavazoie began his oncology fellowship at Memorial Sloan Kettering Cancer Center. The big new thing at that time was precision oncology—the idea that, by rendering a tumor’s genetic fingerprint, it might be possible to tailor drugs and other treatments for individual patients. Yet Tavazoie could never shake the feeling that focusing on the differences, instead of the similarities, among cancers might get in the way of finding broadly acting cures.
His patients’ cancers had sophisticated names, classified into subtypes with technical nomenclature reflecting each cancer’s anatomical birthplace and genetic features. And sure enough, researchers were coming up with increasingly sophisticated ways to attack tumors. Modern oncology had built a standard of care around the singular goal of shrinking the primary tumor. The approach was not wrong per se. It often worked. In some cases, it had resulted in new treatments with spectacular outcomes. The smaller the tumor, the less opportunity for metastasis.
But this school of thought skirted the central problem of cancer medicine rather than addressing it head-on. It is metastasis, not the primary tumor, that kills patients. So why was the focus always on the primary tumor? What we needed, Tavazoie felt, was a better understanding of metastasis.
Now, more than a decade after Tavazoie opened his laboratory, this novel approach is beginning to bear fruit in the form of two promising drug candidates specifically for the treatment and prevention of metastasis. Both drugs are based on an early discovery made by Tavazoie that upended the study of metastasis—that, regardless of what cancer we’re talking about, single genes could strongly drive or suppress metastasis.
The discovery emerged from a series of molecular experiments to separate cells that were capable of metastasis from those that were not. To do this, Tavazoie injected cancer cells into mice and then removed the tumors that formed outside of the initial injection site. After dicing, spinning, and mixing the metastatic tumors, he used traditional techniques to isolate single cells for analysis.
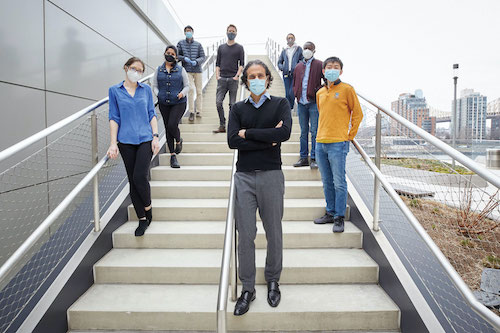
Tavazoie, who is the Leon Hess Professor, knew that there had to be some molecular anomaly that made these metastatic cells unique. But cancer cells are full of genetic anomalies—that’s what makes them cancer cells. Figuring out which specific aberration was allowing these cells to metastasize meant digging through a litany of alterations; some genes were abnormally active, others abnormally inactive. There were lots of clues to why this was the case, but no clear culprit, and certainly no useful information for cancer patients.
“At this point, I wasn’t even thinking of therapies. I just wanted to find genes linked to metastasis,” Tavazoie recalls. “We hoped that what we found might help others, in the future, identify drugs that could target those key genes.”
Eventually, by painstakingly winnowing a long list of candidates, they landed on the specific gene that would end up defining much of the lab’s work and would form the basis of their drug candidates: ApoE.
Tavazoie’s lab, the Elizabeth and Vincent Meyer Laboratory of Systems Cancer Biology, was not the first to look for answers in metastatic cells. As early as 1889, English physician Stephen Paget proposed his “seed in soil” hypothesis based on autopsies of hundreds of women who had died of breast cancer. Paget suggested that metastatic “seeds” spread out from tumors but only form colonies in areas with opportune “soil” for further growth.
“We’re now attacking metastasis from intersecting angles. We have experts in RNA, immunology, metabolism, mitochondrial biology, all focused on the same problems.”
This basic model stood the test of time, and nearly a century later it led to pioneering work by cancer scientist Isaiah Joshua Fidler in the 1970s. Like Tavazoie, Fidler injected cancer cells into mice, monitored for metastasis, and isolated those that managed to spread. Despite lacking the kinds of genetic tools available to Tavazoie, Fidler nevertheless arrived at a high-level picture of metastasis that has endured.
A lot has to happen for a cancer cell to break bad. Most cells in a tumor are perfectly content to remain in place, and, of those cells that do escape, precious few are able to survive. Metastatic cells must change to penetrate healthy tissue or otherwise sneak their way into the circulatory or lymph system. They must develop the ability to fend off immune cells, and, upon arrival in an end organ, they must figure out how to grow their own blood vessels for nourishment.
And it’s not a single alteration that makes a cell capable of the varied tasks required to metastasize. It’s more like a perfect storm of them that accumulate in exactly the right way. Given this reality, it’s remarkable that it happens as often as it does.
Until you consider the possibility that metastasis, like many complex life processes, isn’t actually random.
ApoE was the linchpin,” Tavazoie says.
A well-studied gene, ApoE has been linked to multiple diseases, including Alzheimer’s. But in melanoma cells, Tavazoie found that ApoE was at the center of a suppressive pathway that prevents a cascade of metastatic events from occurring.
The winding road that led Tavazoie and his team to ApoE began with experiments involving microRNA, small segments of RNA that turn specific genes on or off. Since the early 2000s, researchers had known that one of the main features of cancer cells is the total collapse of the microRNA system. Predicting that wayward microRNA might be linked to metastasis, Tavazoie isolated metastatic melanoma cells from mice and scoured the samples for microRNA anomalies, eventually finding a cluster that was entirely out of control.
In metastatic cells, these hyperactive microRNA were shutting down the ApoE gene.
Bit by bit, the mystery of metastasis began to unravel. The Tavazoie lab continued to pursue ApoE, demonstrating that three of the necessities for metastasis—forming new blood vessels, fending off immune cells, and penetrating healthy tissue—were regulated by that one gene. They found that melanoma cells with active ApoE never metastasized and that cells that did spread from the primary tumor invariably had inactive ApoE.
Eventually they came full circle, demonstrating that microRNAs function as a switch to turn ApoE, and metastasis, on and off. Disabling these microRNAs with a cocktail of nucleic acids revived ApoE and prevented metastasis in mice.
“These were very exciting years for us,” Tavazoie says. “We had pinpointed a critical gene that strongly regulates metastasis and could manipulate it to prevent melanoma cancer cells from spreading.”
The discovery of ApoE at the heart of metastasis led to a flurry of activity from the Tavazoie lab, adding more detail to the emerging picture.
One recent Tavazoie lab investigation, spearheaded by Benjamin Ostendorf, a physician-scientist; Jana Bilanovic, a graduate student; and Nneoma Adaku, an M.D.-Ph.D. student, revealed how the three versions of ApoE that exist in humans impact cancer progression differently. They found that ApoE4—a variant that, paradoxically, increases the risk of Alzheimer’s disease—appears to be the most effective subtype when it comes to preventing melanoma metastasis in mice.
A retrospective human study suggested humans benefit from ApoE4 as well. According to medical records of 300 patients with melanoma, those lucky enough to have had the ApoE4 variant were those that survived the longest. This was the first discovery that human hereditary genetics regulate metastasis, and it solved a long-standing conundrum in the field regarding the genetic basis for metastasis.
“We’re now attacking metastasis from intersecting angles,” says Adaku. “We have experts in RNA, immunology, metabolism, mitochondrial biology, all focused on the same problems.”
And the work is now beginning to coalesce around the development of new drugs capable of reviving ApoE. If they are shown to be effective in preventing metastasis in the future, it would be highly impactful for cancer patients. “If we had a therapy that could ensure that the cancer would not spread, a surgeon could take out the primary tumor—and you’re done,” Tavazoie says. “There would be no further treatment, and the cancer would not recur.”
A first attempt at developing such a drug was based on the liver X receptor, which limits the expression of ApoE. Tavazoie and his colleagues showed in 2014 that an oral medication that acts on this receptor prevented metastasis of melanoma in mice. The mechanism was simple and matched years of observations: with the liver X receptor off-line, ApoE was free to produce proteins that block metastasis in healthy cells. One year later, similar techniques led to the identification of another critical metastasis gene that regulates creatine metabolism, and the lab has since discovered a compound that inhibits this gene and prevents colorectal cancer metastasis in mice.
A drug development company co-founded by Tavazoie has begun work on a clinical-grade version of these compounds to test in humans. Phase I trials of the compound, designed to arrest melanoma metastasis, confirm that it activates ApoE in humans just as it had in mice, and clinicians anecdotally report that the compound appears to stabilize the progression of metastasis in many patients with various cancer types. They have also observed examples of metastasis shrinkage responses in multiple patients. A separate drug designed to arrest metastasis in colorectal cancer patients recently began Phase I trials as well.
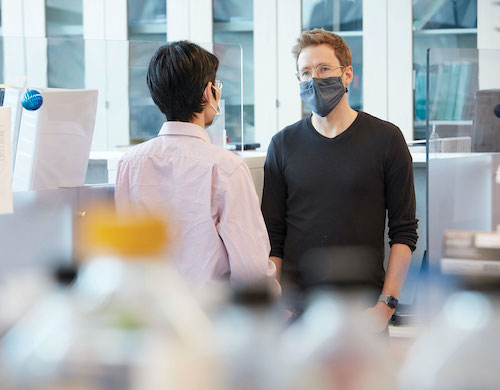
“Both drugs appear to be safe and well-tolerated, and they are providing proofs of concept of what we observed in mice. We’re also excited to hear that clinicians are observing examples of anti-metastatic activity in patients with advanced disease,” Tavazoie says, while cautioning that preliminary reports from early trials designed only to evaluate safety are not scientific claims of efficacy.
Although further trials are in the works, there’s a thorny problem when it comes to launching clinical trials on metastasis drugs. New cancer therapies are typically tested first in patients who are already very sick, for whom more established treatments have failed—it wouldn’t be ethical to deny traditional treatments to people who need them in the hopes that a new drug would work better. But if you wait until those treatments have failed, it’s difficult to evaluate a drug designed to prevent metastasis—such patients already have metastatic cancer. The upshot is that two compounds designed to prevent metastasis from ever happening are being tested in patients who already have advanced metastatic disease.
Nevertheless, Tavazoie is optimistic.
“Metastasis is a real bottleneck event,” he says. “There are millions of cells within a tumor, and only one in 10,000 has what it takes to spread to another organ and form a colony. That we’re up against such an extreme, rare event gives me hope that we will one day learn to prevent it.”
But he does not expect any single drug to spell the imminent end of metastasis. Tavazoie envisions a more prudent future, in which a new generation of anti-metastatic drugs that activate ApoE work alongside existing treatments.
“We don’t intend to replace targeted cancer therapies,” Tavazoie says. “Our work exists parallel to other effective therapies such as hormonal therapies for breast and prostate cancer and immunotherapy for melanoma and lung cancer. Our long-term goal is to find a key pathway that could reduce the likelihood of metastasis by, say, 80 percent. Targeted therapies would extend the patient’s life and remove the cancer, and our therapy would keep it from coming back.”