Feature
Emerging from the age of HIV
First, it was a death sentence. Then, a life sentence. The next step: ridding the world of the virus once and for all.
By Alexander Gelfand. Photography by Allison Michael Orenstein.The aids epidemic in the United States officially began on June 5, 1981. That Friday, the Centers for Disease Control released a routine bulletin to physicians, as it does each week, summarizing public health trends. A short notice on page two reported on five young, otherwise healthy men in Los Angeles who had been hospitalized with very serious cases of pneumonia. CDC scientists thought the coincidence was notable—healthy young men don’t usually get life-threatening forms of the disease.
Within weeks, the CDC had been flooded with calls about unusual infections in young men, many of whom were either gay or IV drug users. A virus was suspected. A task force was created.
At the same time—just six days after the CDC report—on the other side of the country, another young man, Michel C. Nussenzweig, graduated from The Rockefeller University.
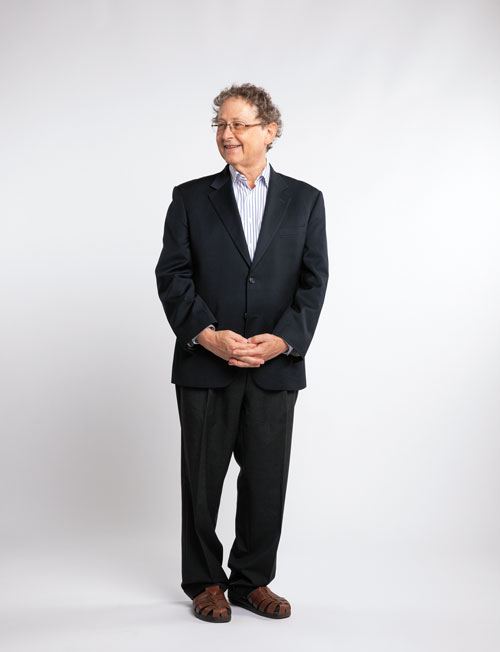
Although he didn’t know it at the time, Nussenzweig’s destiny would become closely intertwined with the virus, which later became known as HIV. In the coming years, AIDS, the disease caused by HIV, would begin ravaging communities in the U.S. and around the world, and some of the best minds in medicine would struggle to bring it under control. Eventually, HIV’s power to enfeeble immune systems would be recognized as one of the gravest health problems of our time—and Nussenzweig would dedicate his career to solving it.
But at the time, he had other plans, and none of them involved HIV.
It was a single, seemingly mundane decision that shifted the course of Nussenzweig’s work.
A physician-scientist, he trained as an immunologist at Rockefeller while attending medical school and went on to a residency in infectious disease in the mid 1980s, a time when the field was shaken by the burgeoning AIDS epidemic. He later returned to Rockefeller to start his own immunology research program. By the turn of the millennium, Nussenzweig was deeply involved in detailed studies of white blood cells called B lymphocytes and the antibodies they produce—molecules that help the immune system recognize invaders, such as bacteria and viruses, as foreigners.
As part of this work, he developed a method for replicating antibody genes that he believed would allow researchers to better understand why immune defenses sometimes fail. And this was where fate, if you believe in that sort of thing, intervened.
He needed a virus on which he could test his new protocol: something that would provoke an immune response and cajole the B cells he was interested in to produce antibodies. He could have chosen anything.
“We had to pick a pathogen, so we picked HIV,” Nussenzweig recalls. “Frankly, I didn’t think it was going to be very interesting.”
But in the years that followed, the antibody response that HIV provoked among Nussenzweig’s B cells, and that he was able to explore using his novel techniques, proved to be interesting in the extreme. Some of these antibodies turned out to have unique and unexpected properties—unexpected enough to prompt Nussenzweig to embark on a steady line of research into the specific interactions between HIV and B cells. By 2008, he had begun to see the possibility of devising new strategies to combat the virus. And before long, much of the activity in his lab was dedicated to that goal: He ran experiments to test those strategies, added clinical researchers to his team, and initiated trials with human subjects.
Although Nussenzweig’s approach is still in its infancy, there’s no denying that it shows tremendous promise. In fact, his work with HIV, along with converging work from several other labs at Rockefeller, may be bringing us closer to an AIDS cure than we have ever been. Their research has breathed new life into a line of inquiry that had once been abandoned in the face of repeated failure: using antibodies to treat, prevent, and possibly even cure HIV infection. And it has led to novel tools for combating other deadly viruses, such as Zika and Ebola, as well.
If antibody therapies live up to their promise, they could become the second landmark success in the history of HIV research. The first occurred in the mid-1990s, when David D. Ho, scientific director of the Aaron Diamond AIDS Research Center (ADARC), a Rockefeller affiliate, established treatments based on multiple antiretroviral drugs to control HIV—a strategy that came to be known as combination, or cocktail, therapy.
“The eighties and early nineties were brutal,” says Martin Markowitz, ADARC’s clinical director. “I’m a gay man, and all my friends were dying of AIDS. I was a doctor by day, a nurse by night, and a mourner by weekend.”
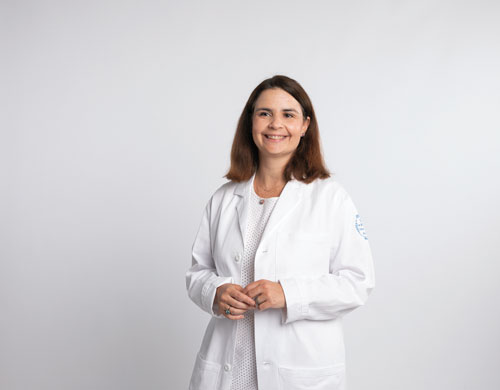
Then, seemingly overnight, the arrival of antiretrovirals transformed the disease from a death sentence to a chronic condition. It gave millions the opportunity to live with the disease rather than perish from it (today 37 million people are HIV-positive worldwide, one million in the U.S.).
“It was a miracle,” Markowitz says.
Over the past few decades, these drugs have only improved. Modern antiretroviral drugs—many of which were first tested at The Rockefeller University Hospital in collaboration with ADARC—can suppress the virus in HIV-positive patients with exceptional efficacy, allowing them to live out a near-normal life span. The drugs are also much less toxic than they used to be, and can even be used to prevent infection in the first place.
But as revolutionary as they are, antiretrovirals are far from perfect. They must be taken in pill form each and every day. They cause side effects ranging from nausea to kidney damage. And, for many of the people who need them most, they’re nowhere to be found.
Huge numbers of people who can and should be taking antiretrovirals aren’t—either because they don’t have access to them or because obtaining them involves stigma or even danger. In some parts of sub-Saharan Africa, for example, which is home to nearly 70 percent of all infected people and where combination therapy can now be had for less than a dollar a day, many women who test positive for the virus are subjected to discrimination and violence. In the U.S., associations between AIDS, homosexuality, and drug abuse have similar consequences. As a result, only 30 percent of HIV-positive individuals around the globe actually receive antiretroviral therapy.
The bottom line: More than 30 years after HIV was identified as the cause of AIDS, there is no vaccine and no way to completely clear the virus from an infected person. Although we’ve come a long way, we still can’t claim victory over the virus.
Typically, it takes the immune system two to three weeks to generate antibodies capable of tackling a virus. But HIV isn’t like other viruses.
For one thing, the virus actively targets the immune system itself. Through various mechanisms—many of them uncovered by Paul Bieniasz, who has spent years exploring how HIV broaches the body’s defenses—the virus invades T lymphocytes, a class of white blood cell that plays a crucial role in organizing the body’s immune response. Evading their built-in antiviral defenses, HIV reprograms the T cells’ DNA, hijacks their internal machinery, and replicates at breakneck speed—killing the infected cells and laying waste to the immune system in the process (see “How HIV attacks,” below).
To make matters worse, HIV mutates at an incredible rate: As soon as the immune system generates an antibody with the ability to neutralize the virus, a new and different strain pops up. That same gift for mutation allows the virus to quickly develop resistance against individual drugs, which is why combinations of multiple antiretroviral medications are needed to keep it in check.
As this cat-and-mouse game continues, the immune system is gradually destroyed, and the patient progresses to full-blown AIDS. Even if the amount of virus in a person’s blood is pushed below detectable levels by antiretroviral therapy, HIV’s ability to integrate itself into the DNA of its host cells allows it to lurk in the body’s tissues. This reservoir of virus represents a lifelong threat: stop treatment, and the infection will come raging back, sometimes stronger than before—a phenomenon known as viral rebound.
Yet curiously enough, occasional cases of HIV are far less menacing. A tiny proportion of infected people—roughly one percent—produce antibodies that neutralize multiple strains of the virus and help the immune system outmaneuver it. But they only begin manufacturing these broadly neutralizing antibodies—which scientists like to call bNAbs, pronounced “bee-nabbs”—after two to four years of infection.
In the late 1990s, researchers explored several ways of using such antibodies, harvested from the lucky one percent, to either treat or prevent HIV. One strategy was to transfer the proteins to test subjects who lacked them with the hope of boosting these individuals’ immune systems—much as modern forms of cancer immunotherapy work by enhancing patients’ ability to kill or suppress their own mutating cells. Another strategy was to develop vaccines that would naturally prompt the immune system to produce broadly neutralizing antibodies in people who are at risk of HIV infection.
But these early attempts failed: The bNAbs that researchers were able to identify, using the techniques available at the time, weren’t potent enough to kill the virus.
For similar reasons, every attempt to develop a vaccine went off the rails.
As a result, researchers largely gave up on antibodies as a means of combating HIV—at least until Nussenzweig’s fateful decision to employ the virus in his B cell experiments caused them to reconsider.
His breakthrough, developed with assistance from collaborators at the National Institutes of Health, was a highly effective means of fishing B cells out of people who had developed broadly neutralizing antibodies, combined with new cloning techniques that made it possible to isolate, analyze, and cultivate generations of highly potent BNAbs. Soon after Nussenzweig’s breakthrough was reported, researchers elsewhere were using his techniques to identify additional bNAbs, and before long scientists throughout the field were diving back into antibody-related research.
After several failed attempts to devise an HIV vaccine, for example, Ho and his team at ADARC abandoned those efforts and instead decided to use the expertise they had gained engineering proteins to construct highly potent bNAbs in the lab.
The researchers have generated a library of close to 250 different antibodies, each with its own unique properties. Last year, they unveiled a custom-built bNAb, developed for preventive purposes, that neutralizes HIV by binding to two different targets: one on the virus, and another on the T cells upon which the virus preys.
Such bispecific antibodies effectively act like two antiviral weapons rolled into one, and this particular model has proven to be especially powerful: When tested in mice, the protein neutralized 99 percent of hundreds of viral strains. “It has exquisite activity against HIV,” says Ho, who recently secured funding from the NIH to develop the antibody for treatment purposes and hopes to begin clinical trials with it next year.
“Our mission is to come up with two bispecific antibodies that could be used as periodic therapy,” he explains, laying out a scenario in which patients could be given an injection every couple of months, rather than consuming a daily menu of antiretroviral pills.
Meanwhile, a different set of clinical trials is already under way with two of the bNAbs that Nussenzweig and his colleagues found in patients. And his lab work has yielded fresh insight into how an HIV vaccine might ultimately be engineered.
In a series of trials conducted at The Rockefeller University Hospital, Nussenzweig and his colleague Marina Caskey demonstrated that the most effective bNAbs they have so far laid hands on—one derived from the blood of an anonymous African woman, the other from an American who has visited Rockefeller to provide samples—can drive the amount of virus in the blood of HIV-positive patients below detectable levels, reducing the risk both of opportunistic infections and of sexual transmission.
Like the antiretroviral drugs that represent the current standard of care, these two antibodies, which go by the unglamorous names 10-1074 and 3BNC117, work best in combination. In work published just this fall in Nature, Caskey and Nussenzweig showed that the two antibodies together, administered three times over six weeks to patients who stopped antiretroviral therapy, suppressed the virus for an average of over five months.
Unlike current antiretroviral drugs, the antibodies continue to provide protection and treatment for weeks after they have been administered.
“And from what we can tell,” says Caskey, “they have no major side effects.”
Nussenzweig and Caskey are now testing versions of the antibodies that have been altered to make them last even longer. Like Ho, they would ideally like to create a single injection that would work for months at a time.
The protection conferred by fully formed antibodies does not last as long as the immunity granted by a vaccine that prompts the body to produce its own. But getting a shot once every few months, rather than taking a pill every day, should go a long way toward mitigating one of the biggest problems with antiretroviral therapy—the fact that many people who initiate the treatment have a difficult time adhering to it. It is a proposition that Nussenzweig and his team will test with a large-scale clinical trial in Africa sometime within the next year or two.
The trial will target young African women, a group that is at once highly vulnerable to infection and unlikely to seek help to prevent or treat it, in part due to the stigma associated with HIV and AIDS. To counter that stigma, trial participants will receive the antibodies in conjunction with a long-acting injectable contraceptive that is already widely accepted by African women.
It is even possible that antibodies could offer a way to address the viral rebound problem.
According to Jeffrey V. Ravetch, the Theresa and Eugene M. Lang Professor and head of the Leonard Wagner Laboratory of Molecular Genetics and Immunology, bNAbs don’t just neutralize HIV itself. They also prompt the immune system to kill human cells infected with the virus, depriving it of a hideout where it might otherwise linger—something antiretroviral drugs cannot do.
“By virtue of their 200 million years of evolution, antibodies are finely tuned to interact with the immune system, not only directly neutralizing a virus but mobilizing the full immune response against it,” he says.
Antibodies owe this superpower to a part of their structure known as the Fc region, which Ravetch has studied extensively in his effort to understand how the immune system functions at large, and how it malfunctions in autoimmune disorders. In fact, he has manipulated the Fc region of Nussenzweig’s broadly neutralizing antibodies to boost their efficacy in mice; and the two are now collaborating with researchers at Caltech and the NIH on ways to further engineer bNAbs to make them even more potent.
Nussenzweig’s tests, meanwhile, have revealed the surprising synergy that can result when bNAbs begin to interact with other immune cells.
In a recent study, he and his NIH collaborators treated monkeys with simian-human immunodeficiency virus, a variant of HIV engineered to infect primates, with 10-1074 and 3BNC117—the same two antibodies that now are being tested in the clinic—for a limited amount of time. The virus initially disappeared from the monkeys’ blood but came surging back several weeks after treatment stopped—similarly to the kind of viral rebound one sees in HIV-positive humans who stop antiretroviral treatment.
Months later, however, something very unexpected happened: Half of the animals spontaneously regained the ability to control their infections. Somehow, they seemed to have overcome their viral rebound-like condition. Further experiments revealed that the antibodies the monkeys received enhanced their immune systems, boosting the response of the same kind of immune cells that prevent some HIV-positive individuals from developing AIDS.
Furthermore, Nussenzweig, Caskey, and Ho are working on strategies to combine bNAbs with a cancer drug called romidepsin, which can wake the virus from its dormant state in a patient’s DNA—kicking it out from its reservoir so that it might be purged from a patient’s immune system completely.
Only time will tell which of these strategies—bispecific bNAbs, Fc-enhanced bNAbs, or combination therapies—will ultimately progress into useful therapies. But even today, it seems reasonable to imagine a not-too-distant future in which HIV could be prevented, treated, and maybe cured with a syringe full of antibodies.
The fruition of broadly neutralizing antibodies into drugs is just one possible scenario. As remarkable as these proteins are, they are not the only game in town (see “How scientists are fighting back,” above).
Just as Nussenzweig and his team are testing long-acting versions of their bNAbs, for example, the researchers at ADARC have been testing injectable antiretroviral drugs that would last longer—and hence be easier for patients to adhere to—than the current daily pills.
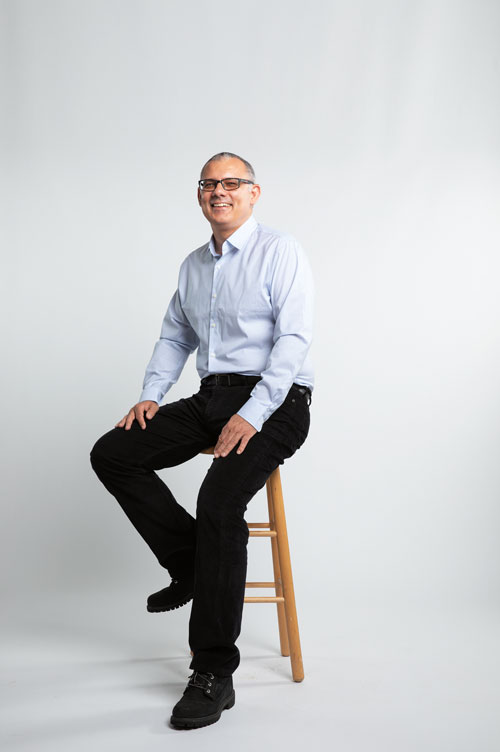
Markowitz recently demonstrated that an experimental drug called cabotegravir suppresses the virus in monkeys, and this effect can be obtained with just one injection every other month. He will soon conduct clinical trials at The Rockefeller University Hospital as part of a large international effort to determine whether cabotegravir and another retroviral drug, rilpivirine, can control HIV infection when administered in combination. And he’s embarking on a project in China to determine whether cabotegravir alone can provide long-lasting protection against the virus.
Unlike antibodies, which can be injected into the fatty tissue that lies just beneath the skin, cabotegravir must be injected deep into muscle, causing discomfort that can last several days—itself a possible obstacle to adherence. But Markowitz recently concluded an animal study showing that another experimental antiretroviral agent, called MK-8591, is so potent that it could conceivably be administered through an implant that releases the drug over a period of several months, eliminating the need for a painful injection.
Meanwhile, Nussenzweig’s work with bnabs has another possible use: as a vaccine. One of the first things he noticed about his broadly neutralizing HIV antibodies was that their genes contained an unusual number of mutations. So many, in fact, that they could not have arisen through the normal process of genetic recombination that B cells ordinarily undergo.
He hypothesized that the process in which the body develops bNAbs must take an especially long time, which would explain why people only begin producing them several years after they get infected. He also supposed it must be an iterative process requiring exposure to many different forms of the virus.
Subsequent studies at Rockefeller and elsewhere confirmed both ideas, and provoked cautious optimism that a workable vaccine—the most distant goal in all of AIDS medicine—is possible.
Just last year, Nussenzweig and his team managed to coax a genetically modified mouse to develop its own bNAbs against HIV, effectively vaccinating the animal. But doing so required injecting no less than nine different bespoke antigens that were prepared with help from colleagues at The Scripps Research Institute. Hence, while their experiment at last offered scientists a blueprint for developing a viable HIV vaccine—a project that draws roughly $1 billion in funding per year, and has thus far produced nothing but disappointment—it also highlighted the challenges involved. Doing something similar in a human would be even more difficult, and no one, including Nussenzweig, expects it to happen anytime soon. Nor would a vaccine that requires so complex a series of inoculations be anyone’s idea of a silver bullet against HIV.
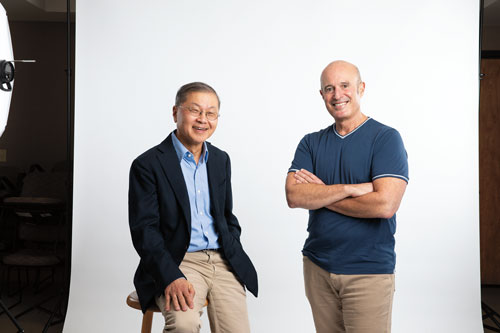
In fact, the path to a vaccine is marred with difficulties—including, somewhat counterintuitively, the introduction of new HIV treatments. Markowitz says the development of long-acting antibodies and antiretroviral drugs could impinge on progress toward a vaccine: For ethical reasons, researchers will never withhold prevention or treatment from potential trial participants, and those who have access to an effective, preventive therapy may be reluctant to give it up in exchange for an experimental one. “The more widespread these treatments become, the more they could complicate the task of enrolling patients in future vaccine trials,” Markowitz says.
Even so, the work being carried out in this area could lead to the development of vaccines for other illnesses.
Earlier this year, researchers in Nussenzweig’s lab and that of Charles M. Rice, the Maurice R. and Corinne P. Greenberg Professor, used Nussenzweig’s methods to isolate an especially effective antibody against the Zika virus, which causes a raft of devastating birth defects. In time, their discovery could lead to new ways of fighting the virus—and might even pave the way to a vaccine.
“Nussenzweig’s work has provoked cautious optimism that a workable vaccine—the most distant goal in all of AIDS medicine—is possible.”
Meanwhile, Paul Bieniasz’s investigations into the HIV virus itself might offer a new way to approach vaccine development in general, much as Nussenzweig’s work has already provided a new paradigm for antibody-related research.
For decades, Bieniasz has studied how HIV enters T cells, replicates, and bursts forth from its diseased and dying hosts. He has identified several mechanisms by which the virus overcomes the antiviral proteins that cells normally use to deal with foreign invaders—mechanisms that could potentially be disrupted by drugs. And together with Theodora Hatziioannou, a research associate professor, Bieniasz has developed a strain of the virus that behaves in a particular species of monkey much the same way as it does in humans—a development that should improve the predictive quality of animal studies.
Recently, however, Bieniasz and his team made a discovery that could have far broader implications.
The researchers found that by adjusting the frequency with which a particular genetic sequence appears in the HIV genome, they could persuade a naturally occurring protein, aptly named ZAP, to recognize the virus’s RNA as foreign and destroy it. By carefully dialing the amount of that sequence up and down, Bieniasz hopes to synthesize viral RNA that can prompt an immune response without causing illness.
If successful, he would be in a position to create an entirely new kind of attenuated virus, stripped of its ability to infect. And that, in turn, could speed the development of vaccines against a whole range of viral diseases, from Zika to Ebola (though not necessarily HIV, whose ability to hack human DNA makes the use of a live attenuated vaccine too dangerous to consider, at least for now).
New forms of prevention and treatment. Novel tools for combating other global scourges. The ultimate goal of developing a vaccine against HIV may still be a ways off, but as David Ho, who has been in this fight longer than most, emphasizes, the gains made in recent years have been remarkable. And what’s to come promises to be even better.
“Science,” he says, “is progressing in this field like no other.”