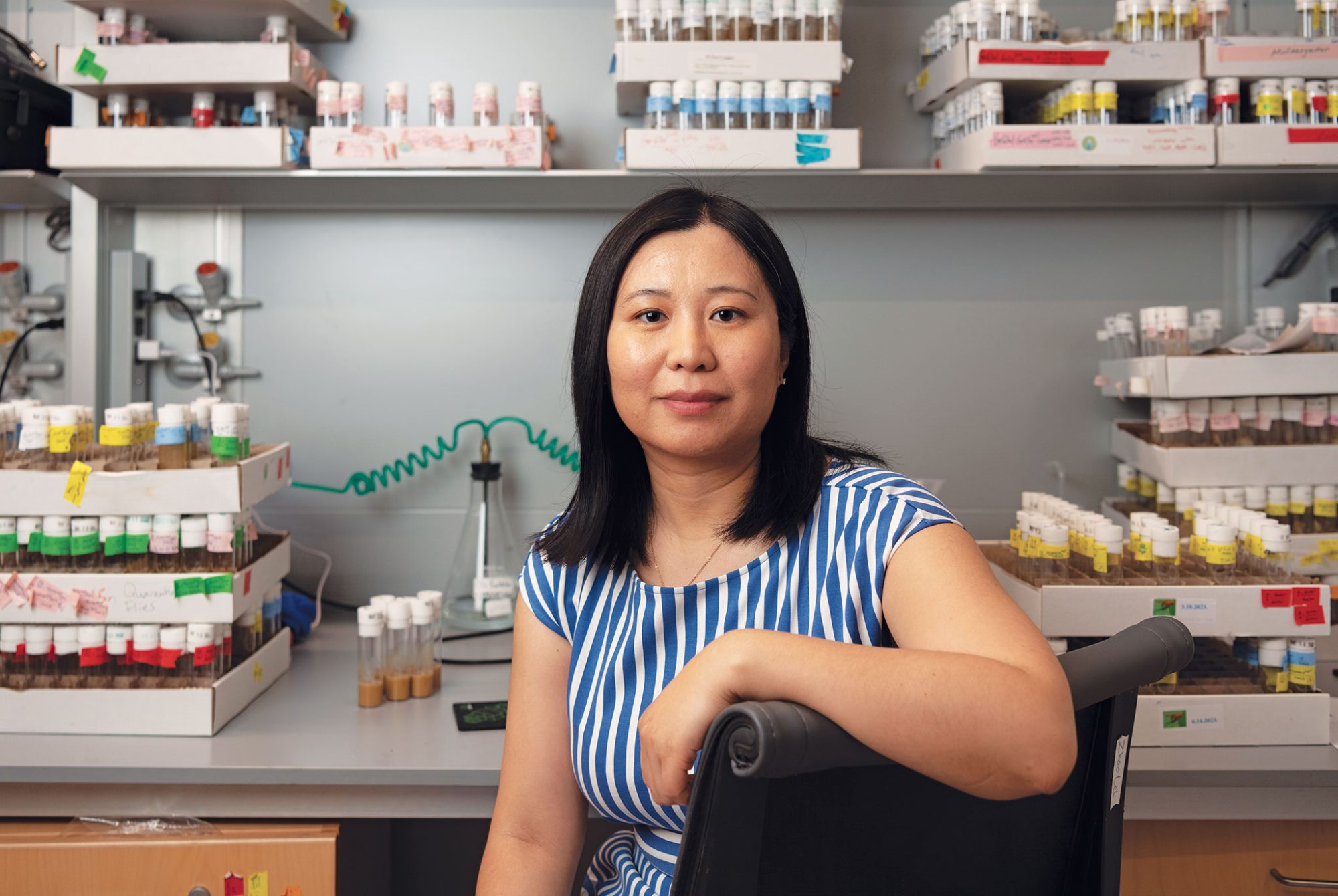
Interview
The rapid evolution of de novo genes
With Li Zhao
In 2006, just a few years after the fruit fly genome had been sequenced, geneticists at the University of California, Davis, made a startling discovery: Several new genes had cropped up, seemingly out of nowhere.
These “de novo genes” weren’t simply new variants of existing ones; they had sprung forth from the supposedly inert spaces in between the coding sections of DNA—regions long dismissed as the junkyards of the double helix. Since the days of Darwin, such sprightly biological change agents had never before been seen.
A young graduate student at the time, Li Zhao was so intrigued that upon graduating in 2011, she set out to join the lab of David Begun, where the discovery was first made. She soon revealed that these little genetic big bangs happen all the time—over the past decade, she and her team have identified more than 500 de novo genes in the Drosophila lineage alone.
But de novo genes are just one of several types of rapidly evolving genes that enable organisms to quickly adapt under intense environmental pressures. Such genes have now been discovered in virtually every organism so studied, including humans.
Since founding Rockefeller’s Laboratory of Evolutionary Genetics and Genomics in 2017, Zhao, an associate professor, has probed deeper into questions about how genes arise, spread, and evolve, shaping individuals and entire species. We asked her to explain what rapid evolution looks like, and how research into its basic mechanisms might expand our understanding of how new life-forms come to be.
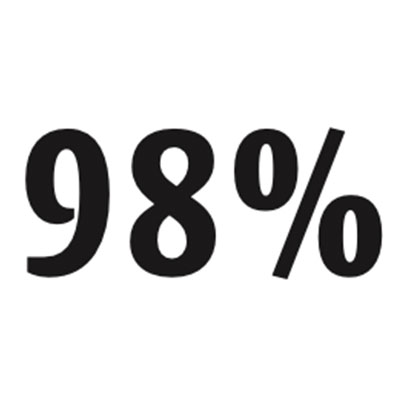
Data
Portion of the human genome that doesn’t code for amino acids, the building blocks of proteins.
What made you jump at the opportunity to study de novo genes?
That genes could build themselves from scratch was just a fascinating idea. When the first ones were found, there had already been hints that genes can evolve very quickly. In the early 2000s, for example, scientists discovered several so-called orphan genes which lacked similarity to genes in other species or lineages. People assumed that these orphans did in fact come from a parent gene, although they had changed so much in a relatively short time span that they looked unique. And in some cases, newly found orphan genes were chalked up to mere sequencing errors.
But I wondered if some orphan genes had in fact emerged in a de novo manner, without a parent. And as I kept learning more and more about de novo genes, I realized that our next challenge would be to understand how they are born—how a supposedly useless piece of DNA turns into an evolutionary asset. It’s a tremendously exciting puzzle to solve.
Can de novo genes show up anywhere in the genome at any time?
Not exactly. We know their origin is mainly related to functions that kick in under very strong evolutionary pressures, like immune functions that get activated during an infection. Organisms are in constant battle with pathogens that lurk in their environment, so genes that can help them keep up with the arms race tend to evolve very quickly.
The same is true for genes that support reproduction. Sexual selection is an incredibly strong pressure, making the male sex organs a hotbed for evolutionary innovation. Any advantage an aspiring father might get from a new gene, such as brighter plumage or hardier sperm, can make all the difference for his reproductive fitness. That’s why my team focused on the testes when we first started looking for rapidly evolving genes in Drosophila.
When you say “rapidly,” in the context of evolutionary change, how rapid is that?
It depends on what genes and what species we’re talking about—the speed of change varies greatly in evolution. On the one hand, many genes governing the most basic biological functions have barely changed since the first life-forms originated on Earth. For example, about a thousand human genes have similar counterparts in bacteria, fungi, and other very distantly related species, which means they come from a common ancestor that lived billions of years ago.
How does a supposedly useless bit of DNA become an evolutionary asset?
On the other hand, you have rapidly evolving genes whose DNA sequences change much more quickly. In Drosophila, we study rapid-evolution events that occurred over up to a few million years. The timescale can be much shorter for humans, however—tens of thousands of years. Many Tibetans, for example, can tolerate high altitudes thanks to mutations in genes playing a role in oxygen use and UV light exposure, an adaptation to the local environment that probably happened only 30,000 years ago.
What does a rapidly evolving gene look like in action?
Let’s stick with UV light exposure, and I’ll give you an example that we’ve recently seen in Drosophila. A senior research associate in my lab, Nicolas Svetec, studied how fruit fly populations in two sites with different exposure, one in Florida and the other in Maine, respond to UV light. When he collected eggs from each location and incubated them under the same UV light, he found that the Florida population survived the exposure at a higher rate. What really grabbed our attention was that the Florida flies also carried unique genetic signatures related to genes that help repair UV-induced DNA damage, suggesting that this population adapted to a sunnier environment by quickly acquiring the ability to counter the effects of UV rays.
What about behavioral adaptations? Do fruit fly mothers in Florida lay their eggs in shadier areas than the Maine mothers do?
That’s a great question, but we have no idea! Drosophila is a wonderful model for studying many genetics-related questions, but we know relatively little about their life outside of the lab.
Have you found any rapidly evolving genes in humans?
Yes, we’ve discovered evolutionarily young genes coding for small proteins of unknown function. Most of these genes are expressed in only a few specialized cell types, such as in heart tissue, and we hope to learn more about them soon.
We’ve also found what look like new genes that are present in cancer cells but absent from normal tissues. We have yet to confirm that these sequences are actually genes, and we still don’t know what proteins they code for. Are they just spurious creations, or are they specifically doing something related to tumor growth or metastasis? We don’t know that either, but it will be fascinating to find out.
What’s next in the field?
Human de novo genes are a very, very hot topic right now. For example, recent work from a group in China found 74 such genes related to brain development, around half of which arose after our lineage branched off from chimpanzees. Although there are controversies around this study, these findings created a lot of excitement as they suggest de novo gene origination might have enabled humans to evolve bigger and more complex brains.
We need to be careful not to draw premature conclusions from such studies, however. This is a brand-new area of research, and it’s very tricky to answer questions about the evolution of human-specific traits. For one thing, the type of experiments we did with the Drosophila eggs under UV light cannot be done in humans—it would involve putting human populations in a controlled environment and following them over decades or centuries! So we need to rely on model species such as Drosophila to delve into the functions and fitness effects of de novo genes.
And while it’s easy to think that humans gained dominance over other primates because our brains are bigger, we actually know very little about what kinds of selective pressure drove the origin and evolution of our species. Are we really smarter and stronger than our primate cousins? And if we are, how exactly did our cleverness give us a leg up in surviving the flu or having children?