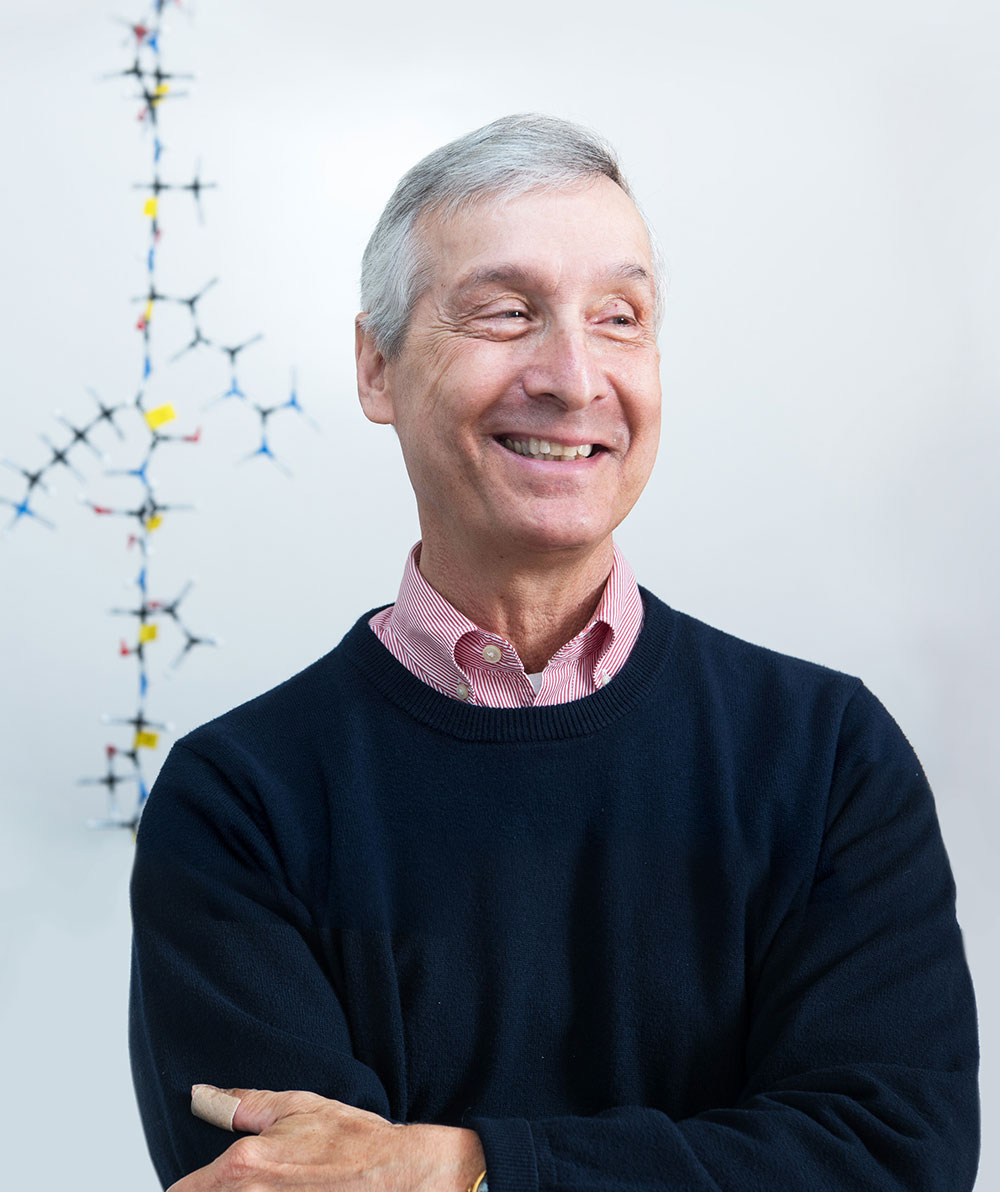
Interview
C. David Allis
Can cancer cells be rehabilitated?
By Eva Kieslermost cancer therapies were developed on the premise that the body must be purged of disease—whether by cutting tumors out or by poisoning them with chemicals or radiation. But tomorrow’s treatments might be based on subtler, more aikido-like solutions.
One such approach is a new class of drugs that, rather than destroy tumors, aims to put cancerous cells on a path back to normalcy and let them lead healthy, productive lives. Such a feat could be possible thanks to epigenetics, a type of indexing system superimposed on DNA. The system exists in all animals and plants, and it ensures every gene gets used precisely the way it should—in the right cell, at the right time, to the right extent.
C. David Allis, a pioneer in epigenetics, says that cancer is often the result of this indexing gone wrong. In many leukemias, for example, certain blood cells that ought to be keeping a low profile instead switch on genes that boost their own growth and survival. In recent work published in Nature, Allis, along with an international team of researchers, presented concepts for drugs that would work by resetting the cells’ internal programming. Their hope is that the approach will be more effective, and less prone to causing side effects, than existing cancer treatments.
We asked Allis, the Joy and Jack Fishman Professor, to tell us more about epigenetic therapy and its promise.
Help us understand what “epigenetic” means and how it’s relevant for cancer.
The word literally means “in addition to” DNA, and it refers to the fact that the DNA in our cells doesn’t exist in isolation, or act on its own. It’s tightly wrapped around spools of proteins called histones, which in fact do a lot more than package DNA—they function like master switches determining what sets of genes in a cell should be active or inactive.
Histones carry an intricate and vast repertoire of chemical marks, which are constantly being modified to turn the expression of genes up or down. In the past few years, we’ve learned that the enzymes responsible for creating or interpreting histone marks are surprisingly often mutated in cancer. In many cases, the disease is the result of both genetic and epigenetic errors acting in concert.
Now that the field is a hotbed for drug discovery, it has taken on a whole new dimension.
There is often not much we can do about classic genetic mutations in which a cell’s DNA sequence has been altered. It’s hard to make drugs to restore those changes. But epigenetic abnormalities are fixable, and potentially reversible, at least on paper. There are now several compounds that target different classes of histone-modifying enzymes, and some are showing promising results in clinical trials.
What can you tell us about the leukemia drug your lab is developing?
Liling Wan, a postdoc in my lab, has discovered that a protein called ENL, together with other proteins, acts as an engine for tumor growth in leukemia cells. ENL is an epigenetic “reader”—its job is to recognize specific marks on histones and activate genes accordingly. More than 35 percent of people with acute myeloid leukemia—and about 70 percent of infants with the disease—have a flawed version of this protein in their blood cells, leading to the faulty activation of cancer-promoting genes.
With support from the Tri-Institutional Therapeutics Discovery Institute, we are now working to develop a compound that can prevent this disease mechanism.
Is the drug working?
We have a lot more work to do before we can make an effective ENL inhibitor and test it in patients. That said, we’re very encouraged by a series of experiments we did in leukemia mouse models. When human AML cells are transplanted into mice, the animals get cancer. But if we remove ENL from these cells by genetic engineering, the cells don’t divide as quickly, and the animals live longer.
Another interesting observation came from work with an experimental leukemia drug that targets Bet, an epigenetic enzyme of the same class as ENL. In our mouse model, we were able to boost the effectiveness of this Bet inhibitor by removing ENL. These findings strengthen our confidence that a well-designed ENL inhibitor could be effective—either by itself or in combination with Bet inhibitors.
Could this potentially work on cancers other than leukemia?
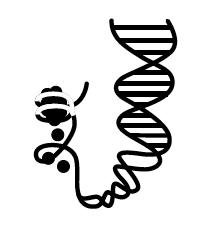
Uncoiled from histones and stretched out, a cell’s DNA would be about 2 meters long.
Yes, and that’s a possibility we’re currently exploring. It turns out that many children with Wilms’ tumor, a rare and often deadly form of kidney cancer, have mutations affecting ENL, leading to the faulty expression of tumor-causing genes similar to those we see in leukemia. Liling and others are now doing experiments on patient-derived Wilms’ tumor cells to see if and how their mutations are linked to epigenetic function.
You started to work on histones when few other scientists were interested, and long before epigenetics became a buzzword. How has the field changed?
In the early 1980s, when my lab first started out, we didn’t even know why histones are chemically modified. It was a hypothesis, originally proposed by Rockefeller biologist Vincent Allfrey in the mid-1960s. And chromatin, the amalgam of DNA and histones, was not nearly as fashionable a topic as it is today. Still, we were possessed to think that both histone-modifying enzymes and the histones themselves are biologically important, seeing that all organisms have them.
It took several decades before we and others began to realize that epigenetic changes can cause cancer, developmental defects, and many other diseases—and before we discovered that these changes are therapeutically pliable. Today, chromatin biology and epigenetics are informing essentially every aspect of biology. Now that the field is a hotbed for drug discovery, it has taken on a whole new dimension.
It’s an incredibly exciting development to be part of.